ABBREVIATIONS
ACC, acetyl-CoA carboxylase; AG, acylglycerols; AMPK, AMP-dependent kinase; BHA, butylated hydroxyanisole; BHT, butylated hydroxytoluene; CD36 receptor, fatty acid translocase; CE, cholesterol esters; Chol, cholesterol; CM, chylomicrons; CMR, chylomicron remnants; DG, diacylglycerols; DM, dry matter; DMEM, Dulbecco’s modified eagle medium; FFA, free fatty acids; LPL, lipoprotein lipase; MDA, malondialdehyde; MG, monoacylglycerols; MTP, microsomal trigliceride transfer protein; pACC, phosphorylated ACC; pAMPK, phosphorylated AMPK; tACC, total ACC; tAMPK, total AMPK; TBA, 2-thiobarbituric acid; TBARS, thiobarbituric acid-reactive substances; TBHQ, tert-butylhydroquinone; TCA, trichloroacetic acid; TEER, transepithelial electrical resistance; TEP, 1,1,3,3-tetraethoxypropane; TG, triacylglycerols.
INTRODUCTION
Synthetic antioxidants, such as butylated hydroxytoluene (BHT), butylated hydroxyanisole (BHA), and tert-butylhydroquinone (TBHQ), are commonly used as antioxidants in the food industry. The use of antioxidants ensures the prolonged freshness of food by stabilising its nutritional value, taste, and colour. Synthetic phenolic antioxidants are primarily used to stabilise frying fats and instant products that have gained popularity among consumers in recent years. Thanks to their ability to quench free radicals, they not only prolong the freshness of food, but also protect consumers from the adverse effects of lipid oxidation products that are important factors in the pathogenesis of atherosclerosis [Plat et al., 2014; Björkhem et al., 1991]. The permitted amount of BHT added to food is 100 mg/kg of fat, while TBHQ and BHA can be used in twice the amount [Commission Regulation (EU) No 1129/2011 of 11 November 2011]. Excessive or improper use of synthetic antioxidants may have carcinogenic effects [Xu et al., 2021].
Although synthetic phenolic antioxidants have been widely used to stabilise dietary lipids for many years, their role in regulating the bioavailability and metabolism of lipids stabilised with them still remains unclear. Data available in the literature indicate that diet supplementation with synthetic antioxidants modifies the metabolism of lipids, affecting their blood profile [Nam et al., 2013; Nishizono et al., 2000; Sun et al., 2020] and body weight of the animals fed [Hung et al., 2019; Nam et al., 2013 Nishizono et al., 2000; Sun et al., 2020; Safer & Al-Nughamish, 1999]. However, these data are scarce and do not show at which stages the changes in lipid metabolism do occur. Moreover, published results are sometimes inconclusive or even contradictory. For example, various effects can be observed not only between antioxidants [Nam et al., 2013; Sun et al., 2020], but also for the same antioxidant tested by different researchers [Hung et al., 2019; Nam et al., 2013; Nishizono et al., 2000]. Studies with rodents, rabbits, and even primates (rhesus macaque) have shown that the addition of BHT or BHA to the diet caused a significant increase in levels of triacylglycerols (TG) and the cholesterol low-density lipoprotein (LDL) and very-low-density lipoprotein (VLDL) fractions in the blood with a concomitant decrease in hepatic TG level [Björkhem et al., 1991; Sun et al., 2020]. In contrast, the addition of TBHQ to animal diets resulted in a decreased blood TG content [Nam et al., 2013; Nishizono et al., 2000]. Using a low dose of TBHQ (0.001–0.0028%) reduced blood TG levels by 22% in diabetic rats [Nam et al., 2013; Nishizono et al., 2000] and in mice fed a high-fat diet [Nam et al., 2013]. It has also been noted that the addition of BHT [Safer & Al-Nughamish, 1999] or BHA [Sun et al., 2020] to the diet in amounts not exceeding 0.1% resulted in higher body weight gains in experimental animals. However, the effect of TBHQ on body weight is not clear and may depend on the species. Administering this antioxidant to mice at 0.001% along with a high-fat diet for 32 days reduced body weight gain by approximately 61% and reduced the amount of lipids stored in adipocytes [Nam et al., 2013]. At the same time, in rats with diabetes mellitus (induced by streptozotocin) that were fed a standard diet with 0.0028% TBHQ for 15 days, an increase in body weight gain was observed even though the animals ate less food than those from the control group [Nishizono et al., 2000]. On the other hand, enriching pig diets with 0.006% TBHQ for 34 days did not affect their body weight gains [Hung et al., 2019].
The bioavailability of lipids from the diet entails digestion in the gastrointestinal (GI) tract followed by intestinal absorption. Digested in the GI tract, triacylglycerols are hydrolysed to free fatty acids (FFA) and monoacylglycerols (MG), which are absorbed by intestinal cells. In enterocytes, the products of lipid digestion are converted back into TG and released into the bloodstream in the form of chylomicrons (CMs). CMs secreted by enterocytes into the bloodstream enable the transport of exogenous TG and exogenous cholesterol [Acevedo-Fani & Singh, 2022]. In addition to TG and cholesterol esters (CE) forming a hydrophobic core, CMs have a hydrophilic envelope that includes apoB48 and apoA-IV. One of the crucial functions of these proteins is to facilitate lipid emulsification in blood plasma. The first of these proteins is considered a CM marker because there is only one apoB48 molecule per chylomicron [Phillips et al., 1997], i.e., more apoB48 determines more CMs. In contrast, the amount of apoA-IV secreted with CM varies, and the regulation of apoA-IV synthesis is unknown. In recent years, apoA-IV has been found to regulate satiety, and a deficiency of this protein has been linked to obesity, arteriosclerosis and diabetes development [Kohan et al., 2013, 2015]. Postprandially, TGs included in CMs undergo lipolysis catalysed by lipoprotein lipase (LPL). LPL activity can be inhibited by apoC-III protein synthesised by liver cells and binding to the CM. The chylomicron remnants (CMRs), formed after TG hydrolysis, are then absorbed by hepatocytes and the apoE protein is involved in the process. The absorption of FFA and CMRs by hepatocytes may affect: (i) CD 36 receptor (fatty acid translocase), which is involved in the absorption of fatty acids by cells, (ii) microsomal triglyceride transfer protein (MTP), which participates in the formation of lipoproteins secreted by enterocytes and hepatocytes into the bloodstream, and (iii) the activity AMP-dependent kinase (AMPK) affecting the intensity of the cell’s uptake of FFA [Harmel et al., 2014]. In addition, AMPK influences lipid metabolism in hepatocytes by controlling the lipogenesis mainly by modifying the activity of acetyl-CoA carboxylase (ACC), a key enzyme of the FA biosynthetic pathway.
Based on the presented analysis of literature data, it can be hypothesised that BHT, BHA and TBHQ, used as antioxidants in high-fat products, can significantly affect both the bioavailability and metabolism of lipids stabilised with them. To verify this hypothesis, we conducted in vitro study entailing: digestion, intestinal absorption (cell line Caco-2) and liver metabolism (cell line Hep G2).
MATERIALS AND METHODS
Materials and reagents
Raw pork fat (no stabilising agent added) was purchased in the company store Szubryt (Chelmiec, Poland), and wheat bread was purchased at the local bakery Pawlak (Cracow, Poland). Aicar, amphotericin B, BHA, BHT, bile salts, Compound C, Dulbecco’s modified eagle medium (DMEM), ethylenedinitrilotetraacetic acid (EDTA), fetal bovine serum, glutamine, glycerol, LPL, pancreatin, phosphate buffered saline (PBS), penicillin, pepsin, protease inhibitor, streptomycin, 2-thiobarbituric acid (TBA), TBHQ, trichloroacetic acid (TCA), 1,1,3,3-tetraethoxypropane (TEP), trypan blue, and β-mercaptoethanol were purchased from Sigma-Aldrich (Saint Louis, MO, USA). Antibodies: anti-apoE, anti-apoA-I, anti-apoA-IV, anti-apoB100, anti-apoB48, anti-CD36, anti-MTP, anti-tACCα, and anti-pACC were purchased from Santa Cruz Biotechnology (Dallas, TX, USA); Grb-2, β-actin and anti-β-actin were purchased from Sigma-Aldrich; anti-apoC-III was purchased from MyBioSource (San Diego, CA, USA); anti-pAMPK was purchased from Cell Signaling Technology (Danvers, MA, USA); and anti-Grb-2 from was purchased from BD Transduction Laboratories (San Francisco, CA, USA). Human colorectal adenocarcinoma ATCC HTB-37 (Caco-2) and hepatocellular carcinoma ATCC HB-8065 (Hep G2) cell lines were purchased from the American Type Culture Collection (Manassas, VA, USA).
Model product preparation
The model high-fat product contained 80 g/100 g wheat bread (dried for 24 h and ground to a grain size of 0.5 mm in diameter) and 20 g/100 g lard rendered from raw pork fat. The ingredients were mixed for 10 min, and the resulting product was divided into four parts. To three of them BHT, BHA and TBHQ were added at 100 mg/kg fat (0.002 g/100 g model product), respectively, and the fourth without antioxidants served as the control. All antioxidants were used in the same dose, but not exceeding the permitted dose in food set in the Commission Regulation (EU) No 1129/2011 of 11 November 2011 on permitted additives. The model product immediately after preparation was used for the in vitro digestion.
In vitro digestion
The procedure of the in vitro digestion described by Minekus et al. [2014] with minor modifications was followed in the study. The scheme of its course is shown in Figure 1. Briefly, the model products (2 g) were placed in screw cap flasks (n=16 for each product), and 1 mL of simulated salivary fluid (SSF: pH 7; 18.8 mM K+; 13.6 mM Na+; 19.5 mM Cl–; 3.7 mM H2PO4–; 13.7 mM HCO3–; 0.15 mM Mg2+; 0.12 mM NH4+; 1.5 mM Ca2+) was added to each. The flasks were shaken for 10 min in a water bath (37°C), and then 2.8 mL of simulated gastric fluid (SGF: 7.8 mM K+; 72.2 mM Na+; 70.2 mM Cl–; 0.9 mM H2PO4–; 25.5 mM HCO3–; 0.1 mM Mg2+; 1 mM NH4+; 0.15 mM Ca2+) was added to each mixture, and the pH of the samples was brought to 2 using 1 M HCl. Simultaneously, a solution of pepsin in SGF was prepared and added to the mixture at a volume of 0.2 mL (12 kU). Pepsin activity in the digestion mixture was 2 kU/mL. Gastric digestion was carried out in a water bath with shaking for 2 h (37°C). Of the 16 replicates intended for gastric digestion, 4 were assigned for the future characterisation of gastric emulsion and 12 for the subsequent digestion step.
The pepsin-catalysed reaction was stopped by adding 5.2 mL of simulated intestinal fluid (SIF: 7.6 mM K+; 123.4 mM Na+; 55.5 mM Cl–; 0.8 mM H2PO4–; 85 mM HCO3–; 0.33 mM Mg2+; 0.6 mM Ca2+) and 1 M NaOH to bring the pH to 7.2. Then, 0.4 mL of bile salts solution (60 mg/g of the model product) was added to the samples, and after thorough mixing, another 0.4 mL of pancreatin solution (4.8 mg/g of the model product) was added. Solutions of bile salts (30%, w/v) and pancreatin (2.4%, w/v) were prepared using the SIF solution. Intestinal digestion was conducted in a shaking water bath (150 swings/min) at 37°C for 2 h. Of the 12 replicates intended for intestinal digestion, 4 were used for duodenal emulsion examination, and 8 for intestinal absorption examination. The mixtures intended for the intestinal absorption examination were centrifuged (10°C, 750×g, 10 min), and the resulting supernatant was stored at –80°C.
Assays of emulsion characteristics
The samples after in vitro digestion under gastric and duodenal conditions were left for 10 min to separate the emulsified layer (the time was determined by the Stokes sedimentation equation) and measure its volume. The emulsification degree was defined as the percentage of the emulsified layer in the total sample volume.
Fat droplets in the emulsion were stained with Sudan III and analysed by microscopy (Zeiss Axio Observer Z1 inverted microscope equipped with digital camera and Zen 2012 software, Carl Zeiss, Jena, Germany). Droplet size was estimated using Lucia measurement software (Laboratory Imagining, Prague, Czechia).
Evaluation of lipid absorption and metabolism in vitro
The design of the procedure for the in vitro evaluation of the absorption and metabolism of model high-fat products after digestion, and the parameters determined at each stage are presented in Figure 1.
Cell lines and culture
Human colorectal adenocarcinoma ATCC HTB-37 (Caco-2) and hepatocellular carcinoma ATCC HB-8065 (Hep G2) cell lines were grown in DMEM, supplemented with 20% fetal bovine serum, 2% glutamine, 10 μg/mL of streptomycin, 100 U/mL of penicillin, and 25 μg/mL of amphotericin B at 37°C in a humidified atmosphere of 5% CO2.
Cytotoxicity assays
Caco-2 cells at 5×104 were seeded onto 24-well plates and, after 24 h of culture, were incubated (2 h) with the BHA, BHT and TBHQ (2 μg/mL). Then, cells present in the fluid and detached from the medium with a 0.1% EDTA solution in PBS were centrifuged (250×g, 5 min, 20°C) and stained with 0.4% trypan blue in 0.81% sodium chloride and 0.06% potassium phosphate. The stained cells were counted in a Bürker chamber. The percentage of dead cells was determined.
Intestinal absorption assays
Caco-2 cells were grown in DMEM in inserts with a pore diameter of 3 μm and a growth area of 0.9 cm2 (Thermo Fisher Scientific, Waltham, MA, USA) at 37°C with 5% CO2 concentration. Transmembrane electrical resistance (TEER; EVOM device with STX2 electrode, WPI company, Sarasota, FL, USA) was measured every other day. The culture was terminated when the membrane was entirely covered with a monolayer of morphologically mature cells, and the TEER reached 200–300 Ω and persisted for two days. Then, the growth medium was removed from the apical and basal compartments, and PBS was added. Cells were incubated for 15 min at 37°C, after which TEER was measured. After the removal of PBS, 0.5 mL of the supernatant obtained after in vitro digestion was placed in the apical chamber. Next, 1 mL of colourless DMEM (1 g/L glucose, no glutamine) was placed in the basal chamber. The experiment duration and final membrane pore size were set at 2 h and 3 μm, respectively, based on preliminary experiments.
At the same time, a study was conducted to determine the effect of AMPK on the secretion of chylomicrons into the the basolateral fluid. To this end, 0.5 mL of the supernatant obtained after in vitro digestion of the model product without antioxidants was applied to the monolayer of Caco-2 cells. Aicar (final concentration of 500 μM) or Compound C (final concentration of 10 μM) was added to the supernatant. Then, 1 mL of colourless DMEM was placed in the basal chamber. Cells (n=8) were incubated for 2 h. In all experiments, the basolateral fluid was collected after the 2-h incubation and stored at –80°C for further analysis. Cells were washed with PBS and incubated in this buffer for 15 min at 37°C; then, TEER was measured. Subsequently, PBS was removed, and the cells were lysed.
Preparation of the lysates of Caco-2 cells and Hep G2 cells
Lysis buffer (50 mM Tris-HCl, pH 7.5, 100 mM NaF, 2 mM EDTA, 10 mM β-mercaptoethanol, 10% glycerol, 5 μg/mL protease inhibitor mixture) was added to the cells in the culture dishes, which were subsequently incubated for 5 min at 4°C. The cells were then transferred to Eppendorf tubes and centrifuged (3,500×g, 5 min, 4°C). The lysates were stored at –80°C for further analysis.
Study of metabolism in hepatocytes – Hep G2 cell model
To this end, 3.5 U/mL lipoprotein lipase was added to 0.5 mL of basolateral fluid obtained after culturing Caco-2 cells and incubated at 37°C for 10 min. The mixture thus obtained was administered to Hep G2 cells growing on 12-well plates with a growth area of 2 cm2 and incubated for 4 h at 37°C (n=4). After incubation, the fluid from wells was collected and stored at –80°C for further analysis. Cells were washed with PBS and then lysed (following the procedure described in subsection “Preparation of the lysates of Caco-2 cells and Hep G2 cells”).
Analytical methods
Western-blot procedure
ApoB100, apoB48, tACC, pACC, apoE, apoA-I, apoA-IV, CD36, MTP, pAMPK, and apoC-III were determined by Western blotting [Mika et al., 2021]. According to the scheme shown in Figure 1, apoB 48 and apoA-IV were determined in the basolateral fluid; pAMPK, CD36 and MTP were determined in Caco-2 cell lysates; apoB48, apoB100, apoA-I, apoC-III and apoE were determined in the fluid obtained after incubation with Hep G2 cells; whereas pAMPK, pACC, tACC and CD36 were determined in Hep G2 cells lysates. Briefly, the samples (50 μg of protein) were mixed with loading buffer (50 mM Tris-HCl, pH 6.8, 0.4% sodium dodecyl sulphate, 10 mM β-mercaptoethanol, 20% glycerol, 0.02 mg/mL bromophenol blue), denatured at 95°C for 5 min and separated either in 6% (for apoB100, apoB48, total-ACCα and phospho-ACC detection), 10% (for apoE, apoA-I, apoA-IV, CD36, MTP and pAMPK detection) or 15% (for apoC-III detection) polyacrylamide gels., Grb-2 and β-actin were used as reference proteins. After electrophoresis and electroblotting, the nitrocellulose membranes were blocked by incubation in a TBS-Tween buffer (10 mM Tris-HCl, pH 7.6, 0.5 M NaCl, 0.5% Tween 20) with 5% non-fat milk for 1 h at room temperature. The primary antibodies (anti-apoE, anti-apoA-I, anti-apoA-IV, anti-apoB100, anti-apoB48, anti-CD36, anti-MTP, anti-tACCα, anti-pACC, anti-apoC-III, anti-pAMPK, anti-Grb-2, and anti-β-actin) were diluted in the same buffer and the blot was incubated for 12 h at 4°C. The dilution of primary antibodies was 1:1,000 and that of secondary antibodies was 1:4,000. After extensive washing in a TBS-Tween buffer, the membranes were incubated with goat anti-rabbit horseradish peroxidase conjugated antibodies for 1 h at room temperature, washed again, and subjected to chemiluminescent signal detection. The protein band intensities were quantified using Freeware image analysis software (ImageJ; https://imagej.nih.gov/ij).
The enzyme-linked immunosorbent assay (ELISA)
The concentration of apoB48 in the basolateral fluid obtained after incubation with Caco-2 cells was also determined by an immunoenzymatic method using an ELISA kit from BioSciences (Allentown, PA, USA). An apoB48 solution (0–188 nM) was used as a standard. The coefficient of variation of the ELISA was <10% (intra-assay) and <12% (inter-assay). ELISA was performed using a microplate reader Model 680 from BioRad (Hercules, CA, USA).
Acylglycerol (AC) assays
The sum of mono-, di- and triacylglycerols was determined using BioSystems lipoprotein lipase/glycerophosphate oxidase/ peroxidase enzymatic assay (Sriperumbudur, Tamil Nadu, India). AC was determined in the supernatant obtained after in vitro digestion, in the basolateral fluid obtained after incubation with Caco-2 cells and in the fluid obtained after incubation with Hep G2. To this end, 10 μL of the test sample was added to 1 mL of the reagent, and the mixture was incubated for 15 min at room temperature. Absorbance was measured at 500 nm wavelength using a flow single beam spectrophotometer Specord 40 (AnalytikJena, Jena, Germany). The TG solution with a concentration of 0–18 μM was used to plot the standard curve.
Cholesterol (Chol) assays
Free and esterified cholesterol content was determined using a cholesterol esterase/cholesterol oxidase/peroxidase enzymatic assay kit from BioSysteme. Cholesterol fractions were determined in: the supernatant obtained after in vitro digestion, the basolateral fluid obtained after incubation with Caco-2 cells, and the fluid obtained after incubation with Hep G2. To this end, 10 μL of the test sample was added to 1 mL of the reagent, and the mixture was incubated for 10 min at room temperature. Absorbance was measured at 500 nm wavelength using a Specord 40 spectrophotometer (AnalytikJena). A Chol solution with a concentration of 0–0.8 μM was used to plot the standard curve.
Free fatty acids (FFA) assays
The concentration of free fatty acids was determined using the enzymatic assay NEFA-HR acyl-CoA synthase/acyl-CoA oxidase/peroxidase kit from Wako Chemicals GmbH (Neuss, Germany). FFA were determined in: the supernatant obtained after in vitro digestion, the basolateral fluid obtained after incubation with Caco-2 cells, the basolateral fluid after incubation with LPL, and the fluid obtained after incubation with Hep G2. To this end, 10 μL of the test sample was added to 0.5 mL of the reagent, and the mixture was incubated for 7 min at 37°C. Absorbance was measured at 546 nm wavelength using Specord 40 spectrophotometer (AnalytikJena). A Wako calibrator (FFA concentration up to 4 mM) was used as a standard.
Thiobarbituric acid reactive substances (TBARS) assays
The supernatant (0.5 mL) obtained after in vitro digestion of the model product was mixed with 0.5 mL of 0.01% (w/v) ethanolic BHT solution (to protect against the formation of peroxidation products during analysis). Next, 0.5 mL of a 10% (w/v) solution of trichloroacetic acid (TCA) in 0.25 M HCl and 0.5 mL of a 0.67% (w/v) solution of thiobarbituric acid (TBA) in 0.25 M HCl were added and the mixture was incubated at 100°C for 20 min. After cooling, the sample was centrifuged (850×g, 15 min, 4°C), and absorbance was measured at 535 nm wavelength (spectrophotometer Specord 40, AnalytikJena). An aqueous solution of TEP with a concentration up to 2 μM malondialdehyde (MDA) was used to prepare a standard curve.
Statistical analysis
Data were expressed as means values ± standard deviation (n=4). Statistical analysis was performed using Statistica 13.3 (StatSoft Polska, Cracow, Poland). One-way ANOVA with post hoc Bonferroni’s test was used to compare groups. Whereas, in order to examine the relationship between the variables, the Pearson correlation coefficient (r) was calculated. In statistical analyses, a level of significance of <5% was considered significant.
RESULTS AND DISCUSSION
Effects of BHT, BHA and TBHQ on in vitro fat digestion
Fat digestion in the human gastrointestinal tract is a process involving emulsification and hydrolysis catalysed by salivary, gastric, and pancreatic lipases. These enzymes work at the interface; hence, lipolysis effectiveness depends, among other things, on the degree of fat emulsification. The effect of synthetic antioxidants on fat emulsification after digestion of a model product under gastric and under duodenal conditions was determined by evaluating the emulsification degree and fat droplet size in the emulsified layer at both stages (Table 1). Some representative images of fat droplets in the emulsion stained with Sudan III are shown in Figure 2. Emulsification degree after gastric digestion ranged from 54.0% to 68.8% and differed significantly (p<0.05) from the control only for BHT (14.7% higher). No significant differences (p≥0.05) were observed in the mean size of droplets forming emulsions (Table 1, Figure 2). However, the leading site of lipid digestion is the duodenum; thus, changes in emulsion characteristics at this stage have the key effect on its hydrolysis. After intestinal digestion, the emulsified layer was already 100% in all samples, including the control, although in the case of BHT, the droplets forming the emulsion had significantly larger diameters compared to the other samples (Table 1, Figure 2). At this stage of in vitro digestion, the favourable changes in emulsion characteristics after gastric digestion observed for BHA were not maintained. The emulsification progress observed between the stomach and duodenum was due to the action of bile salts and advanced TG hydrolysis, and the measured fat droplet diameters were consistent with the values noted in the human gastrointestinal tract [Armand et al., 1999].
Figure 2
Fat droplets stained with Sudan III in the emulsion after in vitro digestion of a model product without (control) and with antioxidants in the stomach (A) and in the duodenum (B). Scale bars were placed on each image. BHT, butylated hydroxytoluene; BHA, butylated hydroxyanisole; TBHQ, tert-butylhydroquinone.
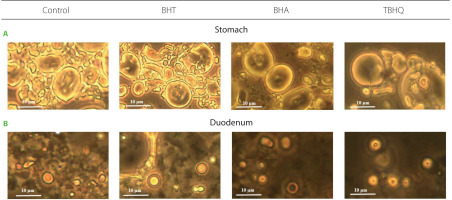
Table 1
Emulsification degree and diameter of fat droplets of model products with antioxidants and without antioxidants (control) after their in vitro digestion under stomach and duodenal conditions, and concentrations of thiobarbituric acid reactive substances (TBARS), free fatty acids (FFA), acylglycerols (AG) and cholesterol (Chol) after digestion under duodenal conditions.
[i] BHT, butylated hydroxytoluene; BHA, butylated hydroxyanisole; TBHQ, tert-butylhydroquinone; TG, triacylglycerols; DG, diacylglycerols; MG, monoacylglycerols; Data are mean ± standard deviation (n=4). Values with different letters within a row differ significantly (post hoc Bonferroni’s test, p<0.05).
Further analyses showed that the synthetic antioxidants did not affect fat digestibility. Indeed, the contents of FFA and AG in the supernatants obtained after in vitro digestion of the model product with or without BHT, BHA and TBHQ did not differ significantly (p≥0.05), (Table 1). Even the addition of BHT did not inhibit fat hydrolysis, although the emulsion formed in its presence had significantly larger droplets. Hence, it can be concluded that pancreatic lipase activity was only to some extent dependent on the degree of substrate emulsification, with fat droplets from 2.1 to 4.2 μm in diameter being hydrolysed with the same strength.
Furthermore, at this stage, the progression of FA peroxidation in the control sample was excluded. No differences were noted in the TBARS content between samples with and without the antioxidants (Table 1). Lipid peroxidation could significantly modify the intestinal absorption of FFA [Courtois et al., 2002].
Effects of synthetic phenolic antioxidants on intestinal absorption
The effect of synthetic phenolic antioxidants on the bioavailability of lipids from an in vitro digested model product stabilised with these antioxidants was examined using the Caco-2 cell line. The selection of Caco-2 cells as the best model for the study of bioavailability was determined by their ability to differentiate into cells morphologically and physiologically similar to normal intestinal cells, i.e. the production of tight connections between each other and the formation of a brush border, the ability to produce enzymes (e.g., alkaline phosphatase, sucrase and aminopeptidase) and systems that transport substances from the lumen of the digestive tract to the bloodstream. The antioxidants were found to be non-toxic to Caco-2 cells under the conditions used; the visibility of the cells was 95.8–97.9% and was not significantly different (p≥0.05) from that of the control (Table 2).
Table 2
Viability of Caco-2 cells, intestinal absorption rate of triacylglycerols (TG) and cholesterol (Chol) of in vitro digested products without (control) and with antioxidants, and the concentration of TG, Chol and apoB48 secreted by Caco-2 cells into basolateral fluid after their treatment with digested model products.
[i] BHT, butylated hydroxytoluene; BHA, butylated hydroxyanisole; TBHQ, tert-butylhydroquinone; AMPK, AMP dependent kinase; Aicar, AMPK activator; Compound C, AMPK inhibitor. Data are mean ± standard deviation (n=4). Values with different letters within a row differ significantly (post hoc Bonferroni’s test, p<0.05).
In the intestinal absorption model, Caco-2 cells take FFA and monoacylglycerols (MG) from the mixture placed in the apical chamber and use them to synthesise TGs that are part of the CMs secreted into the basolateral fluid. In our study, apoB48, apoA-IV, TG, and Chol levels were assayed in the basolateral fluid after incubation of Caco-2 cells with supernatants obtained after in vitro digestion of model hight-fat products. The results are shown in Table 2 and Figure 3A. The addition of antioxidants increased the absorption rate of TG and decreased the absorption rate of Chol. The absorption rates of TG and Chol in the control were 7.0% and 13.8%, respectively, and in the presence of synthetic phenolic antioxidants ranged from 7.8% to 8.5% for TG and from 8.6% to 13.1% for Chol. The antioxidants caused a 22–38% increase in TG secretion by Caco-2 cells compared to the control. BHA also caused a significant (p<0.05) alteration in Chol absorption, reducing its uptake by about 40%. All of the antioxidants also significantly regulated the ability of Caco-2 cells to release apoB48 and thus affected the amount of CMs formed. Most of the apoB48 was released by cells incubated with BHT and TBHQ, 2.1 and 2.6 times more than the cells with the control sample, respectively. These values were also comparable to or greater than those observed when AMPK inhibitor was used. Furthermore, the presence of synthetic antioxidants significantly (p<0.05) affected the amount of apoA-IV released by Caco-2 cells (Figure 3A). In extreme cases, i.e., when incubated with the antioxidants BHT and TBHQ, the concentration in the basolateral fluid was about 90% lower than for the control. Such a significant postprandial decrease in apoA-IV may translate into a lack of satiety after consuming high-fat foods stabilised with these antioxidants. There was a very strong inverse correlation (r=–0.94) between the amount of apoB48 and apoA-IV released by Caco-2 cells. To sum up, the presence of antioxidants significantly modified the structure of CMs secreted by Caco-2 cells. In the case of BHA, the increase in the amount of secreted TGs uncorrelated with the increase in the amount of apoB48 resulted in the formation of less numerous but large CMs additionally stabilised by apoA-IV. In contrast, in the presence of BHT and TBHQ, the CMs formed were more numerous but smaller than in the control sample. This observation, with all proportions maintained, is shown in Figure 4. The scheme shows that for every very large CM released by Caco-2 cells in the presence of BHA, there are as many as 7 and 8 small CMs released in the presence of BHT and TBHQ, respectively, and only 3 intermediate-sized CMs released in the control sample. The importance of apoA-IV in the transport of higher amounts of TG through CM was previously demonstrated in studies performed on porcine intestinal epithelial cells [Lu et al., 2006].
Figure 3
The amount of apoB48 and apoA-IV secreted by Caco-2 cells (A) and levels of microsomal triglyceride transfer protein, MTP (B), phospho-AMP dependent kinase, pAMPK (C) and fatty acid translocase, CD36 (D) in Caco-2 cells after their treatment with digested model products without antioxidants (control) and with butylated hydroxytoluene (BHT), butylated hydroxyanisole (BHA) and tert-butylhydroquinone (TBHQ). Aicar, AMPK activator; Compound C, AMPK inhibitor. Data are mean ± standard deviation (n=4). Significant differences among gropus were marked with different letters (post hoc Bonferroni’s test, p<0.05).
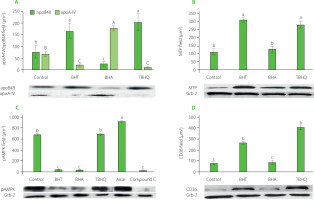
Figure 4
Scheme of the number and size of chylomicrons (CMs) secreted by Caco-2 cells treated with the digested model product without antioxidants (control) and with butylated hydroxytoluene (BHT), butylated hydroxyanisole (BHA) and tert-butylhydroquinone (TBHQ) at 100 mg/kg fat. The amount of CMs was estimated from the amount of apoB48, and their size from the amount of triacylglycerols (grey) and cholesterol (black) per apoB48 molecule.
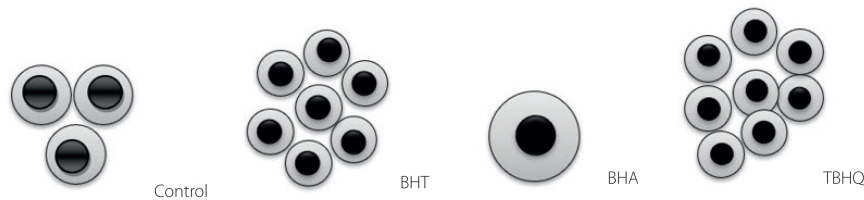
Factors that may contribute to the changes in the composition of secreted CMs are the CD36 transporter located, among others, in the intestinal brush-border membrane and involved in CM formation. Mice with deletion of the CD36 gene secrete less apoB48 [Nassir et al., 2007; Tran et al., 2011]. Another important factor regulating CM synthesis may be the MTP. This protein is located in the endoplasmic reticulum of cells and is primarily responsible for transporting and attaching lipids to apoB48. The absence of MTP occurs in abetalipoproteinemia patients in whom hepatocytes and enterocytes are unable to synthesise lipoprotein B [Burnett et al., 2022]. AMPK, whose metformin-mediated activation has been linked to the inhibition of intestinal absorption of FFA, may also play an essential role in CM synthesis and secretion [Harmel et al., 2014]. The effects of BHT, BHA, and TBHQ on the levels of these proteins in Caco-2 cells are shown in Figure 3 B–D. Similar to Compound C, BHT significantly decreased the amount of pAMPK in Caco-2 cells and simultaneously increased the expression of CD36 and MTP approximately threefold. The ability of Caco-2 cells to synthesise these proteins also increased in the presence of TBHQ, although this antioxidant did not affect the AMPK activity. The BHA worked differently, which decreased the level of pAMPK but did not affect the intensity of CD36 and MTP synthesis by Caco-2 cells.
The juxtaposition of these results with the previously described effects of BHT, BHA, and TBHQ on the ability of Caco-2 cells to release TG and apoB48 (Table 2) showed significant relationships. An inverse correlation (r=−0.72) was observed between the amount of TG secreted by cells and the degree of AMPK phosphorylation. Similar relationships were previously shown for metformin, which enhanced AMPK activity and suppressed intestinal absorption of FFA [Harmel et al., 2014].
A very strong correlation was also found between the amount of apoB48 secreted by Caco-2 cells and the amount of CD36 (r=0.91) and MTP (r=0.99) in cells. The relationship between the amount of CD36 transporter and the amount of CM secreted by enterocytes was observed by Nassir et al. [2007]. However, in their study, decreased amounts of apoB48 were also accompanied by reduced amounts of secreted TG and apoA-IV. In contrast, in our study, BHA caused an increase in the TG uptake and a decrease in the level of CMs, necessitating an increase in apoA-IV synthesis to allow the transport of increased lipids by fewer CMs.
Effects of BHT, BHA and TBHQ on chylomicron catabolism: The Hep G2 cell model
CM catabolism begins with TG hydrolysis catalysed by lipoprotein lipase (LPL) bound to the luminal surface of endothelial cells in capillaries. The FFA released by its action are used by fat tissue and muscles, among others. Effective hydrolysis of TGs transported by CMs depends on the concentration of apoC-III. Due to the presence of hydrophobic residues, this apoprotein exhibits the ability to bind on the surface of lipoproteins and thus restrict LPL access to the substrate [Larsson et al., 2013]. Consequently, the removal of TG-rich CMs from the bloodstream is impaired, and the time of postprandial hypertriglyceridemia is prolonged. In our study, all antioxidants induced enhanced apoC-III secretion by Hep G2 cells (Figure 5A). The greatest increase in the amount of apoC-III (of approximately 70%) was determined for the samples with BHT. For the other antioxidants, the increase in the amount of proteins was about 40%. Due to the study design, the involvement of apoC-III in lipoprotein lipase inhibition was observed. However, in feeding studies, a significant increase in TG concentration (2.5-fold) was observed in the blood of rabbits fed a high-cholesterol diet with 1% BHT [Björkhem et al., 1991]. The authors could not explain the mechanism of the observed changes but suggested that they were related to the inhibition of lipoprotein lipase activity. The involvement of apoC-III in disorders of lipid metabolism has been the subject of many studies. A comparison of apoC-III levels in obese and normal-weight subjects showed that increased body weight is associated with elevated levels of this protein [Talayero et al., 2014]. In addition, high plasma apoC-III levels correlate with the occurrence of coronary artery disease [Onat et al., 2003].
Figure 5
The amount of apoC-III (A), apoE (B), apoA-I (C) and apoB100 (D) secreted by Hep G2 cells and levels of CD36 (E), pAMPK (F) and ACC (G) in Hep G2 cells after their treatment with basolateral fluid obtained after incubation of Caco-2 cells with the supernatant received after in vitro digestion of a model product without (control) and with antioxidants. BHT, butylated hydroxytoluene; BHA, butylated hydroxyanisole; TBHQ, tert-butylhydroquinone; CD36, fatty acid translocase; pAMPK, phospho-AMP dependent kinase; ACC, acetyl-CoA carboxylase. Data are mean ± standard deviation (n=4). Significant differences among gropus were marked with different letters (post hoc Bonferroni’s test, p<0.05).
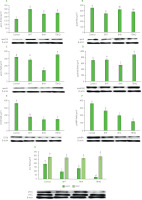
After TG hydrolysis of CMs, CMRs are formed containing mainly CE and apoB48 (apoA-IV is an exchangeable protein and is transferred to HDL). Since there is only one apoB48 molecule per CMR molecule, their size is determined primarily by the amount of cholesterol transported. Caco-2 cells under the influence of BHA secreted the largest CMs containing the highest amount of both TG and CE (Figure 4). Consequently, the resulting CMRs had the largest diameters. In contrast, CMRs in TBHQ and BHT samples were significantly smaller. The efficiency of uptake of the remnants by Hep G2 cells in the control sample was about 63% (Table 3). The smaller CMRs formed in the BHT- and TBHQ-supplemented samples were more efficiently taken up by the cells, and after 4 h of incubation, their absorption rates were 85.0% and 99.5%, respectively. In contrast, the uptake of large CMRs formed in the BHA-containing sample was strongly inhibited, and the amount of particles taken up by the cells reached only 4.4% at the end of incubation. A very strong correlation was found between CMR size and absorption rate (r=0.995).
Table 3
Absorption rate of chylomicron remnants (CMR) and cholesterol (Chol) by Hep G2 cells and concentration of triacylglycerols (TG), free fatty acids (FFA), Chol, endogenous fatty acids (EFA) and endogenous cholesterol (EChol) in the fluid obtained after incubation of Hep G2 cells with digested and treated with Caco-2 cell model products.
CMRs are taken up from the bloodstream by liver cells mainly via membrane-bound LDL receptors [Martins et al., 2000]. It is known that this process requires the presence of apoE, as CMRs cannot bind to LDL receptors via apoB48 because this protein lacks the β2 domain responsible for this process [Hinsdale et al., 2002]. In our study, cells secreted 25% less apoE in the BHT-supplemented sample, but this did not affect the efficiency of CMR removal (Figure 5B).
Efficient CMR uptake by hepatocytes allows cholesterol-rich particles to be removed from the bloodstream. The disruption of this mechanism observed in the samples with BHA may result in the uptake of remnants by macrophages and lead to atherosclerosis [Bentley et al., 2011]. CMRs lack TG, which makes their diameter small enough to get inside macrophages [Proctor & Mamo, 2003]. This can lead to the formation of foam cells that contribute to the development of atherosclerosis [Yu & Mamo, 2000].
In the samples with the addition of the tested antioxidants, Hep G2 cells took up FFA formed after hydrolysis of TGs present in CM more efficiently (Table 3). To identify the mechanism by which synthetic phenolic antioxidants modify the FFA uptake, CD36 receptor and pAMPK levels were examined. Each of the antioxidants tested caused a decrease in CD36 protein content (Figure 5E), as well as in the amount of the active form of AMPK (Figure 5F) in Hep G2 cells. A strong correlation (r=0.833) was found between AMP-dependent kinase activity and the amount of CD36 transporter. Furthermore, strong inverse correlations were determined between FFA uptake and the amount of both pAMPK (r=–0.983) and CD36 transporter (r=–0.919).
Effects of BHT, BHA and TBHQ on lipid metabolism in Hep G2 cells
FFA, derived from dietary lipids, can be used as an energy source (β-oxidation process) or for TG synthesis. TGs and CEs formed in the liver enter into the VLDL or HDL. The marker apoprotein of VLDL is apoB100. For every molecule of VLDL, there is one molecule of apoB100. The primary function of VLDL is to distribute lipids and cholesterol (LDL formed from VLDL after hydrolysis of the TG portion by LPL) into the body’s cells. ApoB100 is a large protein with a molecular weight of 550 kDa [Hegele, 2009]. Within this protein, there is a hydrophobic region that allows the binding of TG and a hydrophilic region that shows an affinity for the aqueous environment of blood. In addition, apoB100 has a binding domain for LDL receptors. This allows the uptake of LDL particles by peripheral tissue cells [Gruffat et al., 1996].
The effect of BHT, BHA and TBHQ on hepatic lipid metabolism was studied using the Hep G2 cell line, which is currently the most frequently used model of human hepatocytes, distinguished from others by its phenotype most similar to the hepatic, e.g., the synthesis of albumin and other proteins, including coagulation factors [Coward et al., 2009]. In the presence of TBHQ, Hep G2 cells secreted significantly more VLDL (apoB100) than the cells of the control sample, whereas BHA contributed to their suppressed secretion (Figure 5D). VLDLtransported CEs are formed from Chol produced in the liver or taken up by hepatocytes as CMR. In our study, a very strong inverse correlation was determined between Chol synthesis in Hep G2 cells and the efficiency of CMR uptake by these cells (r=–0.945). In addition, hepatocytes of the BHA-supplemented sample synthesised almost three times more Chol than the cells of the control samples and nearly 26 times more than the BHT- or TBHQ-supplemented samples (Table 3). Although Caco-2 cells absorbed the least cholesterol in the presence of BHA, the fact that CMR uptake by hepatocytes was completely inhibited resulted in such an intense biosynthesis of Chol that we ultimately determined the highest cholesterol concentration in the culture fluid of Hep G2 cells in these samples. Also, FA synthesis was strongly correlated (r=0.874) with the efficiency of CMR uptake by hepatocytes. Cells in which the CMR uptake was inhibited synthesised less FA (Table 3). Thus, CMR uptake efficiency correlated strongly with the amount of VLDL lipoprotein secreted (r=0.887). Cells that removed most of the CMR under TBHQ treatment secreted the most VLDL.
Of the antioxidants tested, only BHA did not increase TG synthesis in hepatocytes (Table 3). To synthesise TG, hepatocytes use FFA derived from ingested fat and fatty acids produced from non-lipid components. The decrease in hepatic AMPK activation is responsible for inhibiting FA β-oxidation and activating FA and TG biosynthesis [Ford et al., 2015]. Silencing the expression of the gene encoding AMPK in the liver results in increased lipogenesis and increased plasma TG levels [Andreelli et al., 2006]. AMP-dependent kinase controls lipogenesis primarily by altering the degree of phosphorylation of ACC, a key enzyme of the FA biosynthesis pathway. In the case of ACC, the active form of the enzyme is unphosphorylated. All antioxidants tested decreased the amount of the active form of AMPK (Figure 5F) and increased the amount of the active form of ACC (Figure 5G). A strong correlation was determined between ACC activity and the intensity of FA biosynthesis in hepatocytes (r=0.711).
The antioxidants tested also affected the amount of apoA-I synthesised by Hep G2 cells (Figure 5C). ApoA-I is the major structural and functional protein of HDL particles. It accounts for up to 60% of all proteins that make up this lipoprotein and plays a vital role in a process called reverse cholesterol transport. The presence of ApoA-I enables the transfer of cholesterol and phospholipids from cells to HDL particles. This protein attaches to the ATP-A1-binding cassette transporter (ABCA1) located on the cell surface and regulates the function of lecithin-cholesterol acetyltransferase (LCAT), which catalyses cholesterol esterification [Asztalos & Schaefer, 2003]. Reverse cholesterol transport is particularly important in removing excess cholesterol from macrophages and transporting it to the liver. The level of this protein in the fluid after the culture of Hep G2 cells allowed us to evaluate the potential effect of the tested compounds on the production of HDL particles by hepatocytes. TBHQ and BHA significantly (p<0.05) modified the secretion of apoA-I (Figure 5C). For the TBHQ-supplemented sample, higher amounts of apoA-I were determined than in the control sample, whereas for the BHA-supplemented sample, a decrease in the level of this protein was found. In contrast, BHT had no significant (p≥0.05) effect on apoA-I content in Hep G2 cell culture fluid. Therefore, it can be concluded that the use of TBHQ as a food additive can increase HDL fraction cholesterol levels, reducing the risk of atherosclerosis. However, the composition of the apoproteins that make up the HDL fraction plays an essential role in the efficient transport of cholesterol to the liver. Lipoproteins with apoC-III bound on the surface perform less efficiently [Luo et al., 2017]. In human studies, it has been observed that the plasma of patients diagnosed with coronary artery disease has a high content of apoC-III-rich HDL particles [Luo et al., 2017; Riwanto et al., 2013; Xiong et al., 2015]. In our study, synthetic phenolic antioxidants contributed to increased apoC-III secretion (Figure 5A). Hence, stimulating hepatocytes to produce higher apoC-III may result in higher HDL levels that do not protect against atherosclerosis.
CONCLUSIONS
Synthetic phenolic antioxidants (BHT, BHA, or TBHQ) used in the food industry significantly modify the bioavailability and metabolism of lipids. In vitro studies have shown that these antioxidants used at a dose of 100 mg/kg of fat do not affect the digestibility of fat, but induce an increase in the intestinal absorption of TG and modify CM structure. The addition of BHT and TBHQ inhibited apoA-IV synthesis. At the same time, smaller chylomicrons were secreted, but their amount was greater than in the sample without antioxidants. In contrast, BHA activated apoA-IV synthesis, resulting in the formation of fewer but very large CMs. The observed significant decrease in apoA-IV secretion in the BHT- and TBHQ-supplemented samples may result in a lack of satiety after consumption of high-fat foods stabilised with these antioxidants. Also of concern is the increased secretion of apoC-III by hepatocytes observed in the presence of the antioxidants tested, especially with the addition of BHT. Indeed, an increase in LPL inhibitory apoprotein may lead to hyperlipidemia associated with increased CM catabolism time. The efficiency of hepatocyte uptake of CMRs resulting from catabolism was inversely correlated with their size. The small CMRs formed in the samples with BHT and TBHQ were almost entirely taken up, while the uptake of large CMRs present in the samples with BHA by Hep G2 cells was minimal. The prolongation of CMR catabolism time with BHA can lead to hyperlipidemia, the consequences of which include atherosclerosis. Furthermore, the inhibition of CMR uptake in the presence of BHA resulted in a substantial enhancement of cholesterol biosynthesis in hepatocytes. The results of our in vitro study shed new light on synthetic phenolic antioxidants used in food technology in the possible generation of obesity and atherosclerosis. However, at this stage of research, it is impossible to clearly separate antioxidants that promote the development of these diseases from those, which do not do that. To this end, further studies on macrophage cell lines must be carried out to verify CMR uptake, and in vivo studies should be conducted on a properly selected animal model to assess the impact of apoA-IV on the feeling of satiety and food intake.