ABBREVIATIONS
PVA, polyvinyl alcohol; EMA, European Medicine Agency; FDA, United States Food and Drug Administration; GP, grape pomace; GPE, grape pomace extract; TSS, total soluble solids; TA, titratable acidity; TPC, total phenolic content; ABTS, 2,2’-azinobis-3-ethylbenzothiazoline-6-sulfonic acid; DPPH•, 1,1-diphenyl-2-picrylhydrazyl radical, PBS, potassium persulphate solution; TEAC, Trolox-equivalent antioxidant capacity; TAC, total anthocyanin content; TMAB, total aerobic mesophilic bacteria; PCA, plate count agar; PDA, potato dextrose agar.
INTRODUCTION
A third of the world’s food production is lost every year, and about 22% of this loss consists of remnants from fruits and vegetables [Ueda et al., 2022]. Fruits and vegetables are difficult to keep fresh for both markets and consumers because they are easily perishable. Therefore, protection can be provided with the use of edible coatings or other postharvest technologies, which can prevent respiration and oxidation reactions [Duarte & Picone, 2022]. Berries, such as raspberries, blueberries and strawberries have a short shelf life and require appropriate technology to maintain or extend their shelf life [Jafarzadeh et al., 2021].
Strawberry (Fragaria×ananassa) is also called the “queen of fruits” due to its unique taste and flavor and is one of the most consumed fruits worldwide [Riaz et al., 2021]. The worldwide demand for strawberries has increased by more than 80% in recent decades with production exceeding 9 million tons [Kaur & Kumar, 2022]. Strawberries are rich in minerals, dietary fiber, vitamins, flavonoids (including anthocyanins), ellagitannins and proanthocyanidins [Giampieri et al., 2012]; however, their shelf life is limited to 4–5 days under refrigerating conditions [Khodaei et al., 2021]. They feature poor storage potential due to their sensitive structure that is weak to impact, their high respiratory and transpiration rates, and sensitivity to fungal spoilage, especially Botrytis cinerea [Matar et al., 2020]. Freeze-drying and fungicides are widely used to extend the shelf life of strawberries, but these methods are not sustainable because they are costly and cause the formation of residues [Liu et al., 2021].
Edible coatings have been used for food preservation for twelve centuries, and are still of great interest to researchers today due to their protective properties. The term “edible coating” refers to a thin layer of an edible substance that is applied on the surface of food products in the form of a liquid. Its main purpose is to act as a moisture barrier and protect the product from potential harm caused by mechanical damage and chemical reactions [Pavlath & Orts, 2009]. Edible coatings are applied directly on the product surface by spraying, brushing, or dipping to form a modified atmosphere [Armghan Khalid et al., 2022]. Edible coatings are usually biodegradable products that are obtained from various polymers such as proteins, polysaccharides and essential oils and combinations thereof [Riaz et al., 2021]. Active packaging systems with low water vapor and oxygen permeability and the presence of antioxidants and antimicrobials play an important role in minimizing the rate of respiration and maturation, limiting microbial activity and controlling water loss, thereby preserving the freshness of post-harvest crops [Jafarzadeh et al., 2021].
Polyvinyl alcohol (PVA) is a biodegradable, water soluble, edible, non-toxic, film-forming environmentally-friendly polymer with high mechanical properties. Its use in food applications as a packaging and coating material has been approved by the European Medicine Agency (EMA) and the United States Food and Drug Administration (FDA) [Moreira et al., 2020]. It is also relatively inexpensive compared to other synthetic polymers [Ren et al., 2020]. The specific microstructural arrangement of polymeric matrices and the ability to incorporate active ingredients into its structure makes PVA an ideal component for the development of biodegradable active food packaging [Andrade et al., 2021]. Previous studies have reported the use of PVA/chitosan-based coatings to successfully enhance the storage period of strawberries [Ding et al., 2019; Liu et al., 2017]. Moreira et al. [2020] reported that PVA/cashew gum-based coatings could extend the shelf life of strawberries by inhibiting fungal growth.
Natural antimicrobial and antioxidant ingredients are frequently employed in active packaging for food preservation [Parin et al., 2021]. Grape pomace constitutes a major by-product of the winemaking industry. Winemaking by-products and other plant-based agricultural wastes have attracted increasing attention as possible sources of bioactive phenolic compounds in recent years [Makris et al., 2007]. Grape pomace’s phenolic profile includes a wide range of compounds, including proanthocyanins and flavan-3-ols like catechin and epicatechin. Moreover, flavonols such as quercetin, laricitrin, and syringetin, and anthocyanins such as malvidin, delphinidin, petunidin, and peonidin were found [Peixoto et al., 2018] The effectiveness of antibacterial activity is closely related to the presence of phenolic compounds and has been reported effective against both Gram-negative and Gram-positive bacteria [Peixoto et al., 2018].
Our previous work showed that grape pomace extract (GPE) and PVA interacted well and formed films with high elasticity [Kamer et al., 2022]. We also determined that these films have low water vapor and oxygen permeability and good mechanical properties [Kaynarca et al., 2023]. To our knowledge, the combination of grape pomace, which is a cheap agricultural-industrial waste, with PVA for the preservation of quality parameters of strawberries has not been reported to date. In the current study, we aimed to increase the preservation time of strawberries with edible PVA coatings combined with GPE at different concentrations (1, 2, and 2.5%, w/v). First, the grape pomace was extracted and its antifungal properties were investigated. Next, strawberries were coated with PVA/GPE composite using a dipping method. Finally, the quality parameters and microbial loads were examined during strawberry storage.
MATERIAL AND METHODS
Materials
Grape pomace was obtained as Cabernet Sauvignon winemaking waste from a local winery (Vino Desera Winery) in the Kirklareli province of Turkey. Polyvinyl alcohol (PVA) was supplied by Clearity Chemistry Textile Co., Ltd. (Tekirdag, Turkey). Uniformly sized and shaped strawberries (Fragaria×ananassa), exhibiting a surface color intensity of 80% or greater, were procured from a local supplier in Tekirdag, Turkey, soon after being harvested. The strawberries were placed in a cool box after picking and delivered to the laboratory within 1 h. Botrytis cinerea12c, a pathogenic fungus, was obtained from the culture collection of the Department of Plant Protection at Tekirdag Namik Kemal University, Turkey.
Extraction of grape pomace
Grape pomace (GP) was first ground to enable easier extraction, and then incubated with a 1:5 (w/v) solution of 70% (v/v) ethanol in an orbital shaker (INFORS HT Ecotron, Bottmingen-Basel Switzerland) at 25°C for 48 h [Kaynarca et al., 2022]. The liquids were filtered through paper and the solvent was evaporated in a rotary evaporator (RE 100-PRO, SCI LOGEX, Rocky Hill, CT, USA) at 50–55°C. The extracts of grape pomace were dissolved in distilled water and the solutions were stored at –18°C until use. Our previous research [Kaynarca et al., 2022] details the chemical and mineral composition of GPE, including its phenolic compound contents.
Preparation and formulation of PVA/GPE coating solutions
A solution of polyvinyl alcohol (PVA) with a concentration of 5.0% (w/v) was prepared through the dissolution of PVA powder in distilled water at 80°C for 2 h while being stirred. The solution was subsequently cooled to a temperature of approximately 50°C. Aqueous solution of GPE was employed to create a more uniform blend. The dry matter content of GPE was determined to be 16.92%. GPE was added to the PVA solution at concentrations of 1%, 2%, and 2.5% of dry weight of the extract by volume of the solution, and the mixture was stirred for 10 min until it became homogenous. The mixture was allowed to cool to 25°C before being applied to the strawberries. Coating solutions with added GPE were coded as GPV1, GPV2, GPV2.5, and control, based on the varying mass ratios of GPE.
Application of coating to strawberries
The strawberries underwent a washing process for a duration of 3 min using cool, sterile, distilled water, followed by a subsequent 30-min drying period at a temperature of 20°C. Strawberries that were immersed only in distilled water for 3 min and then left to dry served as the control sample. The other groups of strawberries, labeled as GPV1, GPV2, and GPV2.5, were dipped in a PVA/GPE solution containing varying concentrations of GPE (1%, 2%, and 2.5% (w/v), respectively) for 2 min. After coating, the strawberry fruits were drained and dried for 2 h. To perform physicochemical properties, bioactive compound contents, and microbiological analyses of strawberries with different coating applications, 5 strawberries were selected for each analysis set. The strawberries were divided into 5 packages, with each package containing 5 strawberries for analysis on days 0, 4, 8, 12, and 16. This process was repeated three times to conduct the three sets of analyses for each coating application. All strawberries were stored in plastic containers measuring 16×9×5 cm and were refrigerated at 4°C throughout the 16-day analysis period.
Determination of physicochemical characteristics of strawberries
Weight loss
Strawberries were weighed on a digital balance (Isolab, Eschau, Germany) on the first day of the experiment after coating and on 4, 8, 12, and 16 day through the storage period. The percentage of weight loss relative to the initial weight was calculated with Equation 1:
where: wi is the weight measured on the initial day and wf is the weight measured on different days of storage.Total soluble solid content, titratable acidity, and pH
Total soluble solid (TSS) content of strawberry fruit juice was determined at 20°C using a digital refractometer (ATAGO, Tokyo, Japan), with results represented in %. The titratable acidity (TA) was calculated by extracting 10 g fruit in 50 mL of distilled water and titrating the solution with 0.1 M sodium hydroxide until it reached a final pH of 8.1. TA was represented as percentage (%) of citric acid. The acidity of the fruit was measured by a pH meter (HANNA HI2002, HANNA Instruments, Woonsocket, RI, USA). For the chemical analysis, 5 randomly chosen fruits (with a total approximate mass of 30 g) were homogenized with a high-speed mixer and each sample was tested three times.
Texture parameters
The hardness of the strawberries was measured with a texture analyzer (TA-HD plus, Stable Micro Systems, Godalming, Surrey, UK) with a P/36R probe and a crosshead speed of 5 mm/min. Two sets of measurements were made for each replicate on opposite sides of the central zone [Ding et al., 2019]. The values were expressed as Newton (N). Five different strawberries were analyzed for each treatment, and results were given as the mean value.
Color parameter analysis
The skin color of strawberries was measured using a CR-400 Chroma Meter (Konica Minolta Inc., Tokyo, Japan). The chromaticity parameters L*, a*, and b* were used to describe the lightness, the greenness/redness, and the blueness/yellowness, respectively.
Determination of bioactive compound contents and antioxidant activity of strawberries
Ascorbic acid
Strawberries were tested at different times during storage for their ascorbic acid content using the 2,6-dichlorophenol-indophenol (0.25 g/L) titrimetric assay [Vahid, 2012]. Each mL of 2,6-dichlorophenol-indophenol solution was equivalent to 0.2 mg ascorbic acid. Results were expressed as mg of l-ascorbic acid per 100 g of strawberries.
Total phenolic content
Total phenolic content (TPC) was evaluated using the FolinCiocalteu reaction. Briefly, strawberries were blended at 1:20 (w/v) with 80% (v/v) methanol and extracted at 25°C for 24 h. Next, 500 μL of Folin-Ciocalteu reagent were added to 100 μL of each sample. After 2 min, 1 mL of 75 g/L Na2CO3 was added, followed by 94 mL of distilled water [Pineli et al., 2011]. After 60 min of incubation in the dark, the absorbance was measured at 760 nm with a Shimadzu 02910 spectrophotometer (Shimadzu, Kyoto, Japan). Pure gallic acid was used to generate a standard curve, and the results were calculated as g of gallic acid equivalents (GAE) per kg on a fresh weight basis (g GAE/kg).
Antioxidant activity
Antioxidant activity was evaluated using the 2,2’-azino-bis(3-ethylbenzothiazoline-6-sulfonic) acid radical cation (ABTS•+) and 1,1-diphenyl-2-picrylhydrazyl radical (DPPH•). Antioxidant activities of the uncoated control fruits and the coated fruits were determined on the first day and throughout the storage period. The extracts of the samples obtained as described above were added to 600 μL of a methanolic solution of DPPH radical (1 mM). The mixture was incubated in the dark for 30 min and the absorbance was measured at 517 nm. The radical scavenging activity was expressed as IC50(as the amount of extract that scavenges 50% of the DPPH radicals, mg/L of reaction mixture) [Yildirim-Yalçin et al., 2022].
The ABTS assay was conducted as described by Re et al. [1999] with some modifications. A stock solution was generated with 7 mM of ABTS in phosphate buffer solution (PBS) with 2.45 mM of potassium persulphate solution (K2S2O8) and then allowed to stand at 25°C in the dark for 15–16 h. PBS was used to dilute the stock solution to a final absorbance value of 0.700±0.05.
A Trolox calibration curve was used to calculate Trolox-equivalent antioxidant capacity (TEAC) values expressed as μmol Trolox equivalent per kg fresh weight (μmol Trolox/kg).
Total anthocyanin content
The total anthocyanin content (TAC) of strawberries was determined by the pH differential method as described previously [Wrolstad, 1993]. To this end, anthocyanins from the strawberries were extracted using 2% HCl in methanol at a fruit to solvent ratio of 1:5 (w/v) for 60 min. The supernatant was taken and filtered through a 0.45 μm filter. The residue left behind in the filter was re-extracted until a clear solution was obtained. The pH of the diluted fruit extracts was adjusted to 1.0 and 4.5 using potassium chloride buffer and sodium acetate buffer, respectively. The absorbance of the diluted samples was recorded at 522 (A522) and 700 nm (A700) using a Shimadzu 02910 spectrophotometer and A value was calculated with Equation 2:
The TAC of the strawberries was calculated on the basis of a molar absorptivity (ε) of 22,400 L/(mol×cm) and molecular weight (MW) of 433.2 g/mol of pelargonidin 3-glucoside (Pg3G) using Equation 3:
where: DF, dilution factor; L, pathlength (L=1 cm).Microbiological analyses
The total aerobic mesophilic bacteria (TMAB) and total yeast and mold contents for each treatment were analyzed after 0, 4, 8, 12, and 16 days of storage using the spread plate method. Strawberries were homogenized using a Stomacher (Interscience, Saint Nom la Brétèche, France) in a Stomacher bag and then diluted in sterile peptone water. TMAB count was determined after 48 h of incubation at 30°C with the surface plate method on plate count agar (PCA, Merck, Darmstadt, Germany). Yeast and mold counts were determined on potato dextrose agar (PDA, Merck) after incubation for 5 days at 25°C. The microbiological counts of strawberries were expressed as log colony forming units (cfu) per g of sample. Three replicates were carried out for each treatment and storage duration.
Determination of bioactive compound contents and functional properties of the coating solution
The total anthocyanin content, antioxidant activity, and total phenolic content of coating solutions were evaluated using the methods detailed in the section titled “Determination of bioactive compound contents and antioxidant activities of strawberries.”
The antifungal activity of coating solutions with 1%, 2% and 2.5% GPE (w/v) was determined according to the method of Bouchra et al. [2003] with some modifications. To this end, coating solutions were prepared with ethanol to a final concentration of 2,000 mg/L and then mixed with sterile PDA to obtain final concentrations of 0, 20, 80, 100, 120, and 200 mg/L. B. cinerea was first cultured on PDA at 25°C. Petri dishes were inoculated with a mycelial plug (6 mm) of 7-day-old culture of B. cinerea. Three replicates on individual Petri dishes were carried out for each treatment. Ethanol was used as a negative control. After 48 h of incubation at 22°C, the inhibition zones were measured. Results of antifungal activity were expressed as IC50 (concentration that reduced mycelial growth by 50%) determined by regressing the inhibition of radial growth values (percent control) [Mendoza et al., 2013].
Statistical analysis
All data were statistically analyzed using the SPSS, version 18.0 (SPSS Inc., Chicago, IL, USA). Data was subjected to analysis of variance (ANOVA). Tukey’s post hoc test was used to compare the differences in data means. Differences at the level of p<0.05 were considered as significant. Experiments were carried out in triplicate.
RESULTS AND DISCUSSION
Physicochemical characteristics of strawberries
Weight loss
Strawberries with PVA/GPE coating and the uncoated control samples both showed a successive weight loss during storage (Figure 1A). The processes of respiration, sweating, and oxidation can cause fruits to lose weight while they are being stored [Ayranci & Tunc, 2003]. Notable differences were found between the control and coated fruits throughout the storage period (Figure 1A). A comparison of the three coatings revealed that the GPV2.5 coating provided the best protection with only 8.26% weight loss during storage after 16 days, compared to 15.03% loss in the control fruits. Lower GPE concentrations of the coating solution were less effective at preventing weight loss. The use of coatings is evidently useful in providing a physical barrier against moisture loss, thereby delaying dehydration and shriveling of the fruit [Fawole et al., 2020].
Figure 1
The weight loss, total soluble solid content, pH and titratable acidity of coated by dipping in solution of polyvinyl alcohol with 1%, 2% and 2.5% (w/v) grape pomace extract (GPV1, GPV2 and GPV2.5, respectively) and uncoated (control) strawberries during storage at 4°C for 16 days.
Values are given as mean and standard deviation. Different capital letters represent the difference between treatments, different lowercase letters represent the difference between storage times (p<0.05).
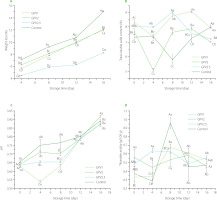
Total soluble solid content, titratable acidity, and pH
The total soluble solids content of GPV1 and GPV2 samples decreased during the first 4 days of storage, but increased afterwards (Figure 1B). This could be an indication of the transition from optimal ripeness. The initial TSS level was 7.4% in the control fruits and ranged from 7.8 to 8.4% in the coated fruits. It is possible that the presence of water-soluble dry matter in the GPE contributed to the higher initial TSS value obtained with coated strawberries compared to the control group. In contrast to the loss may have increased the TSS level [Cordenunsi et al., 2005]. After the 12th day of storage, the TSS content of coated strawberries was reduced (Figure 1B). It most likely resulted from a total degradation of the fruits. Significant differences in the TSS levels were identified between the three different coatings (p<0.05).
The pH values of the coated and uncoated strawberries are shown in Figure 1C. The pH of strawberries increased significantly during storage (p<0.05). This was expected since organic acids are used for respiration during storage of the fruit [Mohammadi et al., 2021]. No significant differences (p≥0.05) in the pH values were observed on the first day of storage between coated and uncoated fruits; however, significant differences (p<0.05) in pH values were observed on the subsequent days of storage with the different coatings.
During the initial 4 days of strawberry storage, a statistically significant reduction (p<0.05) in total acidity (TA) was observed in the untreated control group. However, on day 8, all the samples, except GPV2.5, showed an increase in TA levels (Figure 1D). This initial reduction in the TA until day 4 may be attributable to the conversion of acid into sugar due to ongoing respiration in the fruit [Amal et al., 2010]. Significant (p<0.05) changes were seen in the TA as a function of duration of storage and between the samples (Figure 1D). The application of GPE coatings minimized the intensity of acidity decrease during storage, possibly due to the lower respiration rates of coated fruit compared to uncoated fruit. Our study results are consistent with the findings of earlier studies [Petriccione et al., 2015; Rasouli et al., 2019].
Texture parameters
The firmness of both coated and uncoated control fruits decreased with storage (Figure 2A). The decrease in the firmness of fruit during storage in the current study was found to be consistent with the findings reported in available literature on PVA-coated fruits [Ding et al., 2019]. As strawberries ripen, they can lose their firmness significantly over time due to the hydrolysis and depolymerization of pectin contributing to the deterioration of the middle lamella of the cell wall and loss in turgor [Riaz et al., 2021]. Softening of strawberries due to such water loss and deterioration of cell walls can lead to microbial growth [Sayyari et al., 2022]. The PVA/GPE coated fruit showed significantly (p<0.05) higher firmness compared to the control fruit (Figure 2A). The firmness of the samples at the beginning of storage was in the range of 18.25–18.93 N. On day 16 of storage, the firmness decreased to 3.81–8.99 N. Nonetheless, the PVA/GPE-coated samples showed a lower reduction in firmness than the uncoated ones. Thus, while a 55% decrease in firmness was observed with the uncoated samples on day 4, a comparable decrease was observed in the GPV1 and GPV2.5 samples on day 16 of storage. The uncoated samples showed a 75% decrease in firmness at the end of the storage period while the GPV2.5 sample showed a 51% decrease in firmness making it the sample with the least decrease.
Figure 2
The firmness and color parameters (L*, a*, and b*) of coated by dipping in solution of polyvinyl alcohol with 1%, 2% and 2.5% (w/v) grape pomace extract (GPV1, GPV2 and GPV2.5, respectively) and uncoated (control) strawberries during storage at 4°C for 16 days. Values are given as mean and standard deviation. Different capital letters represent the difference between treatments, different lowercase letters represent the difference between storage times (p<0.05).
Values are given as mean and standard deviation. Different capital letters represent the difference between treatments, different lowercase letters represent the difference between storage times (p<0.05).
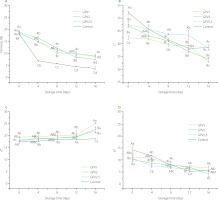
Color of strawberries
Similarly to firmness, color is an important parameter that defines consumer acceptability of fruits. Photographs showing the color and general appearance of the strawberries taken during their storage are given in Figure 3. Considering the color parameters, in general, the L* value determined for both coated and uncoated strawberries decreased significantly (p<0.05) during storage (Figure 2B). The initial L* values of 52.15, 42.46, 47.28, and 47.50 in control, GPV1, GPV2, and GPV2.5 samples, respectively, decreased to 38.53, 33.46, 34.04, and 38.20 on day 16, respectively. However, the GPV2.5 samples showed more or less constant L* values until day 12 of storage. The decrease in L* value is consistent with a darkening of the fruit during storage, as reported previously [Ding et al., 2019; Sayyari et al., 2022]. The redness values of the coated samples remained stable until day 12 and increased afterwards (Figure 2C). There was no change (p<0.05) in the a* values between the coated samples until day 12 of storage. The b* value showed a significant (p<0.05), albeit modest decrease during storage in both coated and uncoated samples (Figure 2D).
Bioactive compound contents and antioxidant activity of strawberries
Ascorbic acid
The initial ascorbic acid content of the PVA/GPE-coated strawberries was significantly (p<0.05) higher than that of the control uncoated samples (Figure 4A). Thus, while the initial ascorbic acid content of the control strawberries was 212 mg/100 g, the same for GPV1, GPV2, and GPV2.5 were 311 mg/100 g, 355 mg/100 g, and 365 mg/100 g, respectively. During storage, the ascorbic acid content of both coated and uncoated control fruit showed a decrease. At the end of day 16 of storage, the control group showed a 75.58% decrease in ascorbic acid content, whereas the decreases for coated fruits were 74.39%, 57.10%, and 59.14% in the case of GPV1, GPV2, and GPV2.5 coatings, respectively. Thus, the loss of ascorbic acid was considerably low with the use of both GPV2 and GPV2.5. Such a decrease in strawberries with edible coatings may be attributed to a decrease in respiration, which can delay ascorbic acid oxidation [Amal et al., 2010]. These findings are in agreement with Gol et al. [2013], who reported that the ascorbic acid content in 1% hydroxypropylmethyl cellulose (HPMC) + 1% chitosan (CH) coated strawberries was significantly higher than in the control samples.
Figure 4
Ascorbic acid and total anthocyanin contents of coated by dipping in solution of polyvinyl alcohol with 1%, 2% and 2.5% (w/v) grape pomace extract (GPV1, GPV2 and GPV2.5, respectively) and uncoated (control) strawberries during storage at 4°C for 16 days.
Values are given as mean and standard deviation. Different capital letters represent the difference between treatments, different lowercase letters represent the difference between storage times (p<0.05).
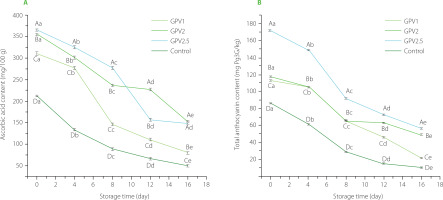
Total phenolic content
Strawberries are an important source of bioactive ingredients, but adverse storage conditions can reduce their quality [Tahir et al., 2018]. The total phenolic content of the strawberries ranged from 94 to 358 g GAE/kgin all samples during storage (Table 1). A statistically significant (p<0.05) decrease in the TPC of both coated and uncoated samples was observed during the storage period. On day 4 of storage, the TPC decreased by 24% and 6% in the control and GPV2.5 samples, respectively. Until day 12 of storage, the TPC was the highest in the GPV2.5 sample, followed by the GPV2 and GPV1, respectively. The preservation of the phenolic compounds of the fruit was clearly the greater, the higher was the content of GPE used in the coating material. The control strawberries had the lowest TPC. The decline in TPC observed towards the end of the fruit storage duration could be attributed to the disintegration of cell structure as the fruit undergoes senescence [Macheix et al., 1990]. TPC may have also decreased due to oxidation processes [Ali et al, 2019]. The application of PVA/GPE coatings appears to have slowed down the oxidation of phenolic compounds, resulting in their preservation. Yan et al. [2019] and Gol et al. [2013] have conducted research that yielded comparable findings, demonstrating the beneficial impact of edible coatings enriched with chitosan (CH) and carboxymethyl cellulose (CMC) on TPC in strawberries.
Table 1
The total phenolic content (TPC) and antioxidant activity determined as Trolox equivalent antioxidant capacity (TAEC) in ABTS assay and as IC50 of DPPH assay of coated by dipping in solution of polyvinyl alcohol with 1%, 2% and 2.5% (w/v) grape pomace extract (GPV1, GPV2 and GPV2.5, respectively) and uncoated (control) strawberries during storage at 4°C.
Antioxidant activity
The antioxidant activity of the strawberries was significantly (p<0.05) enhanced by coating them with PVA/GPE (Table 1). The GPV2.5 coated samples showed 2,591 μmol Trolox/kg in ABTS assay and IC50 of 4.60 mg/L in DPPH assay, giving the highest antioxidant activity among all samples, as expected. This was likely contributed by the high antioxidant activity of grape pomace itself [Rockenbach et al., 2011]. The antioxidant activity of all strawberry samples significantly (p<0.05) decreased over time during storage (Table 1). However, the PVA/GPE coating resulted in greater retention of the antioxidant activity over longer periods of time. On day 16 of storage, the antioxidant activity of GPV2.5-coated fruit was 1238 μmol Trolox/kg (ABTS assay) and 16.49 mg/L (IC50 of DPPH assay), whereas respective values determined for the control samples were 1,028 μmol Trolox/kg and 30.55 mg/L, respectively. These findings are consistent with a previous study by Petriccione et al. [2015] who reported that chitosan-coated strawberries retained higher antioxidant activity after cold storage compared to uncoated strawberries. Due to its functional qualities, the presence of the GPE in the coating not only increased the antioxidant content, but also served as a barrier to oxygen during the coating process, further retaining antioxidant activity.
Total anthocyanin content
Anthocyanins, which are responsible for the red color of strawberries, determine the degree of ripening and the attractiveness of the strawberries [Khodaei & Hamidi-Esfahani, 2019]. A decrease in TAC was observed during the storage of strawberries (Figure 4). This decrease in anthocyanin content could be due to aging and decay of the fruit [Khodaei & Hamidi-Esfahani, 2019]. Similar outcomes have been reported for strawberries stored in the refrigerator [Khodaei & Hamidi-Esfahani, 2019; Sayyari et al., 2022]. In the current study, the use of edible coatings containing different amounts of GPE was effective in preventing the deterioration of anthocyanins in strawberries during storage. The TAC of the uncoated samples decreased from an initial value of 86.33 mg Pg3G/kg to 11.75 mg Pg3G/kg at the end of storage. GPE is an anthocyanin-rich resource [Rockenbach et al., 2011]. Therefore, as expected, the anthocyanin content of the GPV2.5-coated strawberries was approximately two-fold higher than of the control uncoated fruit. The TAC of the GPV2.5-coated fruit decreased from 171.57 mg Pg3G kg to 56.62 mg Pg3G/kg during storage. These values reflect a decrease in the TAC value of 87% in the control sample and 66% in the GPV2.5 sample. The oxygen barrier formed by GPE coating most likely contributed towards the protection of anthocyanin content.
Effect of PVA/GPE coating on microbial growth
The total mesophilic aerobic bacteria (TMAB) and yeast/mold counts of strawberries uncoated and coated with PVA/GPA during the storage period are shown in Figure 5. The initial TMAB count of strawberries was approximately 2 log cfu/g. During storage, the TMAB count increased in all samples, but this increase was seen earlier in the control uncoated samples compared to the coated samples. The uncoated control samples also had significantly (p<0.05) higher TMAB counts compared to the coated samples after 8 days of storage. After day 16, the TMAB content of the control, GPV1, GPV2, and GPV2.5 samples was 9.80, 8.01, 7.00, and 6.67 log cfu/g, respectively. The TMAB count of the GPV2.5-coated strawberries remained constant for the first 8 days, which is likely to be due to the antibacterial effect of GPE. The mesophilic bacteria count increased after day 8 in all GPE-coated samples. A statistically significant increase in yeast/mold count was seen in all strawberry samples (p<0.05) during storage. The initial yeast/mold count of control, GPV1, GPV2, and GPV2.5 samples was 2.68, 2.78, 2.60, and 2.56 log cfu/g, which increased to 14.00, 12.06, 11.57, and 10.45 log cfu/g at the end of storage, respectively. The coated samples showed stable yeast/mold counts between 8-12 days of storage, while the TMAB count showed an increase. Grape pomace has a known antimicrobial effect against many bacteria [Hassan et al., 2019]. In addition, extracts with phenolics obtained from mixtures of Cabernet Sauvignon, Carmenere and Syrah grapes have been reported to have a high inhibitory effect against the mycelial growth of the phytopathogenic fungus B. cinerea [Mendoza et al., 2013].
Figure 5
Total mesophilic aerobic bacteria count and yeast/mold count of coated by dipping in solution of polyvinyl alcohol with 1%, 2% and 2.5% (w/v) grape pomace extract (GPV1, GPV2 and GPV2.5, respectively) and uncoated (control) strawberries during storage at 4°C for 16 days.
Values are given as means and standard deviation. Different capital letters represent the difference between treatments, different lowercase letters represent the difference between storage times (p<0.05).
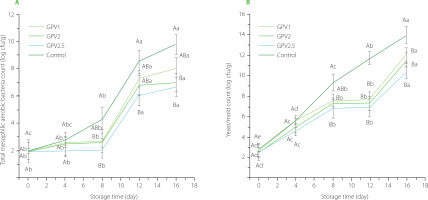
Bioactive compound content and functional properties of coating solution
Coating solutions of PVA with 1%, 2%, and 2.5% GPE (w/v) were tested for their total phenolic content, antioxidant activity, total anthocyanin content, and antifungal activity against B. cinerea. The PVA/GPE solutions demonstrated antioxidant activity, which was positively correlated with the concentration of GPE in solution (Table 2). Thus, GPV2.5 showed the highest TEAC (181 μmol Trolox/kg) compared to GPV2 (152 μmol Trolox/kg) and GPV1 (138 μmol Trolox/kg). The IC50 values of DPPH assay ranged from 37.57 mg/mL (GPV2.5) to 81.55 mg/mL (GPV1). The total phenolic content of the coating solutions was increased in direct proportion to the amount of GPE added. TPC was 263, 475, and 519 g GAE/kg for GPV1, GPV2, and GPV2.5, respectively. We have previously reported that GPE is rich in phenolic compounds such as myricetin, gallic acid, quercetin, ellagic acid, syringic acid, epicatechin, chlorogenic acid, catechin and caffeic acid [Kaynarca et al., 2022]. Anthocyanins are remarkable pigments with red, orange, pink and blue colors, and the most common are cyanidin, pelargonidine, delphinidine, petunidine, peonidine, and malvidin [Zhao et al., 2022]. Grape pomace left after wine production is an abundant and inexpensive source of anthocyanins. Grape pomace extracts are primarily composed of malvidin 3-glucoside, followed by peonidine 3-glucoside, along with other components such as petunidin 3-glucoside, delphinidine 3-glucoside, and cyanidin 3-glucoside [Lapornik et al., 2005]. As expected, the highest anthocyanin content was determined in GPV2.5 containing 85.00 mg Pg3G/ kg while GPV1 had the lowest anthocyanin content of 22.00 mg Pg3G/kg.
Table 2
Total phenolic content (TPC), antioxidant activity determined as Trolox equivalent antioxidant capacity (TAEC) in ABTS assay and as IC50 of DPPH assay, total anthocyanin content (TAC), and antifungal activity against Botrytis cinerea of the coating solutions of polyvinyl alcohol with 1%, 2% and 2.5% (w/v) grape pomace extract (GPV1, GPV2 and GPV2.5, respectively).
Depending on environmental factors, 80-85% of strawberries perish shortly after harvest due to the presence of gray mold (B. cinerea) [Hernandez-Munoz et al., 2008]. Therefore, the antifungal activity of the coating solutions against growth of B. cinerea was determined in our study and results are shown in Table 1. The IC50 values were found to be 48.19, 38.32, and 26.33 mg/L for GPV1, GPV2, and GPV2.5, respectively. The addition of grape pomace had an obvious antifungal effect, and this effect was more robust at higher concentrations of the pomace (p<0.05). Such an antifungal effect of PVA/GPE solutions as well as the findings from other microbiological analyses of stored strawberries suggest that coated with PVA/GPE fruits may be stored for longer periods of time compared to uncoated strawberries.
CONCLUSIONS
PVA/GPE coating solutions, which have high antioxidant activity and phenolic compound content as well as antimicrobial and antifungal properties, could delay the decay of strawberries during storage and prolong their shelf life. The use of coating solutions of PVA with 2.5% (w/v) GPA turned out to be the most effective approach to preserve the quality of strawberries as they ensured a significant delay in weight loss and ascorbic acid content and had positive effects on the preservation of higher contents of total phenolics and total anthocyanins. PVA/GPE coating could be used to provide an edible coating with antifungal effects against B. cinerea. Overall, the current study shows that extending the shelf life of strawberries by coating them with PVA/ GPE solutions may contribute to reducing food loss in the post-harvest chain both during distribution and household storage.