ABBREVIATIONS
AUC, area under the curve; DF, dietary fiber; DPPH•, 2,2-diphenyl-1-picrylhydrazyl radical; FFAR2, free fatty acid receptor 2; FFAR3, free fatty acid receptor 3; GIP, glucose-dependent insulinotropic polypeptide; GLP-1, glucagon-like peptide-1; GPCR, G protein-coupled receptors; ITT, insulin tolerance test; MeOH, methanol; OGTT, oral glucose tolerance test; PC, positive control; PDF, Pithecellobium dulce (Roxb.) Benth. fruit flour; PSS, physiological saline solution; PYY, peptide tyrosine-tyrosine; RS, resistant starch; SCFA, short-chain fatty acids; T2DM, type 2 diabetes mellitus; UC, unmanipulated control; VC, vehicle control.
INTRODUCTION
The promotion of foods with bioactive properties has become a crucial strategy for maintaining health and reducing the risk of metabolic diseases. Functional foods, particularly those rich in dietary fiber (DF) and phenolic compounds, have garnered significant attention due to their demonstrated benefits on key metabolic processes [Adefegha, 2018]. These bioactive compounds contribute to glycemic control, enhance insulin sensitivity, and support intestinal health through mechanisms such as modulation of gut microbiota, reduction of oxidative stress, and regulation of inflammatory pathways. As diet plays a fundamental role in preventing and managing chronic diseases, encouraging the inclusion of these functional components in daily nutrition represents a promising approach to improving overall metabolic health and quality of life [Mondal et al., 2024].
Within this context, Pithecellobium dulce (Roxb.) Benth. stands out as a promising source of these bioactive components. Native to Mexico and also found in South America, Africa, and Southeast Asia, P. dulce has a long history of traditional use in food, medicine, and as animal forage [Wall-Medrano et al., 2016]. The fruit of this legume consists of sweet arils surrounding black seeds, and its nutritional profile is particularly notable for its dietary fiber content (~6 g/100 g) and phenolic compounds [Pio-León et al., 2013]. The red arils are rich in anthocyanins, such as pelargonidin 3-O-glucoside and cyanidin 3-O-glucoside, which contribute to its antioxidant and metabolic effects [López-Angulo et al., 2018]. However, despite the fruit’s availability in Mexico, its consumption remains limited due to the seasonal nature of its maturation, as it is produced only once a year [Wall-Medrano et al., 2016].
The health benefits of DF and phenolic compounds are supported by well-documented mechanisms. DF improves glycemic control by enhancing viscosity and undergoing fermentation, a process that generates short-chain fatty acids (SCFAs). These SCFAs activate free fatty acid receptors 2 and 3 (FFAR2/FFAR3) and stimulate the secretion of anorexigenic hormones, such as glucagon-like peptide-1 (GLP-1) and peptide tyrosine-tyrosine (PYY), which regulate appetite, glucose homeostasis, and insulin sensitivity [Dalile et al., 2019]. On the other hand, phenolic compounds exert complementary mechanisms that contribute to glycemic regulation and metabolic health. These include the inhibition of key digestive enzymes, such as α-amylase and α-glucosidase, which modulate carbohydrate digestion and postprandial glucose levels. Additionally, phenolic compounds enhance glucose uptake through the activation of glucose transporter type 4 (GLUT-4) and peroxisome proliferator-activated receptor-γ (PPAR-γ), as well as reduce oxidative stress and protect pancreatic beta cells from damage, supporting overall glucose homeostasis [Kang et al., 2020]. Since 90% of phenolics pass into the colon, they influence the gut microbiota, which can transform them into bioactive, low-molecular-weight phenolic metabolites [Gowd et al., 2019]. These metabolites help regulate metabolic networks, favoring the growth of SCFAs-producing bacteria like propionate and butyrate, which enhance insulin sensitivity and reduce inflammation [Wang et al., 2022]. These effects position phenolics as key compounds in dietary strategies to prevent and manage metabolic disorders.
Given its distinctive nutritional and phytochemical profile, P. dulce may act as a functional food with the potential to support metabolic health. While its antioxidant and hypoglycemic properties have been observed in previous in vitro and in vivo studies [López-Angulo et al., 2018; Pradeepa et al., 2013], the impact of P. dulce on glucose homeostasis and SCFA production in biological models remains unexplored. This study seeks to fill this gap by evaluating the administration of P. dulce flour (PDF) on SCFA production and glucose metabolic responses in BALB/c mice, providing valuable insights into its potential application for the management of metabolic diseases.
MATERIALS AND METHODS
Plant material
Pithecellobium dulce (Roxb.) Benth. fruit was harvested between March and April 2022 in the town of Autlan de Navarro, Jalisco, Mexico (19.7682200° N, 104.3666400° W). The peel was removed from the arils, which were then placed in 500 g hermetic bags and frozen at −18°C until use. To obtain the flour, the arils were thawed, and the seeds were manually removed. They were then washed with distilled water, excess water was drained, and the arils were ground in a food processor until a crushed paste was obtained. This paste was spread on trays and dehydrated in an oven (DESH304/032014, Todumex, Guadalajara City, Mexico) at 50°C for 8 h. The dried arils were ground in the food processor until fine flour and then passed through a No. 200 mesh sieve (<74 µm). The PDF was packaged in airtight, vacuum-sealed bags to preserve its stability and extend shelf life.
Chemicals and reagents
Folin-Ciocalteu reagent, gallic acid, catechin, vanillin, 2,2-diphenyl-1-picrylhydrazyl radicals (DPPH•), methanol, ethanol, chicory inulin (I2255), n-butanol, total DF assay kit (TDF100A),and citric acid were purchased from Sigma-Aldrich (St. Louis, MO, USA). All other chemicals were of analytical grade.
Proximate chemical analysis
To further investigate the potential of PDF as a functional food, its Proximate chemical composition (ashes, crude protein, and total lipids) was analyzed using standard AOAC International methods [AOAC, 1995]. Moisture content was determined by Norma Mexicana regulation [NMX-F-083-1986]. The DF content determination was performed with Sigma-Aldrich total DF assay kit, separating the soluble from the insoluble fiber fractions. Total carbohydrates were calculated by difference. Total reducing sugars were determined based on Norma Official Mexicana regulation [NOM-086-SSA1-1994].The results of the analyses were expressed on a PDF dry matter (dm) basis. The kilocalories expressed per 100 g of dry matter were calculated using the conversion factors for fruits (proteins 3.36 kcal/g, carbohydrates 3.60 kcal/g, and fat 8.37 kcal/g) due to their similarity in the nutritional content of fruits and not legumes acc. to P. dulce classification [FAO, 2003].
The pectin content of the PDF was determined using the Carré & Haynes [1922] method. PDF (5 g) was added to 100 mL of distilled water and boiled for 15 min on three occasions. After each boiling, the mixture was filtered, and the volume was adjusted to 400 mL by adding 100 mL of water. The filtrate was treated with 100 mL of 0.1 M NaOH and left to rest for 12 h. Then, 50 mL of 1 M acetic acid was added, followed by 50 mL of 1 M calcium chloride, with resting for 1 h. The mixture was boiled for 5 min and filtered. The residue was washed with 500 mL of hot distilled water, re-dissolved in 100 mL of distilled water at 25°C, and boiled for 5 min. Finally, it was filtered through previously weighed open-pore filter paper, washed, and dried until a constant weight was achieved. Pectin content in PDF dm (g/100 g dm) was calculated using Equation (1), based on the weights of the residue (w1), paper (w2), and sample (w3):
Analysis of phenolic compounds
Extraction
Based on the method described by Mattila & Kumpulainen [2002], with adaptations, 2 g of PDF were extracted using 7 mL of 80% methanol with 10% acetic acid (85:15, v/v). The mixture underwent 30 min of sonication (1510R-MT, Brandsonic, Connecticut, USA) followed by centrifugation at 560×g for 10 min (Z326 HERMLE Labortechnik GmbH, Wehingen, Germany). The residue was re-extracted using the same procedure. The pooled extracts were analyzed for total phenolic content, total flavonoid content, tannin content and antioxidant capacity.
Total phenolic content
The total phenolic content was quantified using the Folin-Ciocalteu reagent [Singleton & Rossi, 1965]. A mixture consisting of 3 mL of deionized water, 50 µL of extract, and 250 µL of Folin-Ciocalteu reagent was prepared. Following 8 min, 750 μL of a 20% Na2CO3 solution was added, and deionized water was used to bring the volume up to 950 μL. Using a UV-vis spectrophotometer (UNICO S-2150, Dayton, NJ, USA), the absorbance was measured at 765 nm. A calibration curve was constructed using gallic acid as the standard, with 13 points ranging from 0 to 1,000 mg gallic acid/L. The results were expressed as mg of gallic acid equivalents in 100 g of PDF (mg GAE/100 g).
Total flavonoid content
A catechin solution of 25 mL was prepared by dissolving 0.0250 g of catechin in 80% (v/v) methanol to obtain a calibration curve. Each catechin dilution or extract (1,000 µL), 4,000 μL of distilled water and 300 μL of 5% NaNO2 were mixed. After 5 min, 300 μL of 10% AlCl3 was added and 1 min later 2 mL of 1 M NaOH and 2.4 mL of distilled water were added to complete the volume to 10 mL. Each tube was shaken for 30 s, and absorbance was measured at 415 nm [Moo-Huchin et al., 2015]. Calibration curves were constructed using catechin with concentrations ranging from 0 to 1,000 µg catechin/mL. The results were expressed in mg catechin equivalents in 100 g of PDF (mg CE/100 g).
Tannin content
The tannin content of PDF was determined with minor modifications based on the methodology outlined by Broadhurst & Jones [1978]. A calibration curve was generated using catechin (0.02655 g) dissolved in 80% (v/v) methanol, with concentrations ranging from 0 to 1,000 µg catechin/mL. In test tubes, 50 μL of extract, 3.0 mL of 4% vanillin solution in methanol, and 1.5 mL of HCl were combined. The mixture was thoroughly shaken and allowed to rest for 15 min. Absorbance was measured at 500 nm, and the results were expressed as mg CE/100 g of PDF.
Antioxidant capacity
The antioxidant capacity of PDF was evaluated using the DPPH assay [Brand-Williams et al., 1995], with modifications. Trolox was used for the standard curve (1.97 to 11.75 µg/mL). Absorbance of reaction mixtures was measured at 517 nm, after 30 min of incubation in darkness. The DPPH radical scavenging activity was expressed as µg of Trolox equivalents (TE) per g of PDF dm.
In vivo assays
Animal model and experimental design
The Institutional Committee for the Care and Use of Laboratory Animals (CICUAL) of the Centro Universitario de Ciencias Exactas e Ingienerias de la Universidad de Guadalajara (CUCEI-UDG) accepted the animal experimentation protocol (approval opinion: 02/2022). Healthy male mice of the BALB/c strain (7 weeks of age with an approximate weight of 21–26 g) were purchased from the Vivarium of the Universidad Autonoma del Estado de Hidalgo (Pachuca, Hidalgo, Mexico). They were transported to the experimental animal room of Pharmacobiology Department from CUCEI/UDG. Mice were acclimatized for 2 weeks before starting the experimental process, in a controlled environment (12 h light-dark cycle, temperature: 22°C) with a standard pellet diet (Purina brand Nutri-cubos, Mexico City, Mexico) and drinking water ad libitum. The nutritional content of the diet is shown in Table S1 in Supplementary Materials. Animal experimentation was conducted by the protocols outlined in the NOM regulation [NOM-062-ZOO-1999]. Four groups of 10 mice each were randomly assigned after they were weighed: unmanipulated control (UC) group, vehicle control (VC) group, positive control with inulin (PC) group and PDF group.
Dose standardization
A preliminary test was conducted to determine the most effective dosages of inulin and PDF during the first intervention phase. To prevent precipitation and improve sample dissolution, the sample water solutions were ultrasonicated in bath for 10 min (Sonicator Lab-Tech Instrumentation S.A. de C.V., Mexico).
Based on concentrations of 6.25, 12.50, and 25.00 mg/kg for PDF and 6.25, 25.00, and 50.00 mg/kg for chicory inulin, dose-response curves were generated using the oral glucose tolerance test (OGTT). A vortex (G560, Scientific Industries Inc, Bohemia, NY, USA) was used to shake the samples before each administration. Using an orogastric cannula, the dosages were given orally (v. o.). Before OGTT, mice were fasted for 4 h. Using an Accu-Check Active glucometer (ROCHE, Basil, Switzerland) blood was drawn by a tail puncture at the following intervals to test blood glucose (mg/dL): −30, 0, 15, 45, 60, 90 and 120 min. The different doses tested were administered orally at a time of 30 min.
Prolonged administration
The second stage of the experiment began after the animals had been acclimated for two weeks to routine feeding in a controlled setting (22°C, 12-h light/dark cycles). The VC, PC and PDF groups received the appropriate solution orally. The VC group was administered physiological saline solution (SSF), the PC group received reconstituted inulin at a concentration of 50 mg/kg, and the PDF group was given a solution containing 6.25 mg/kg of PDF, dissolved in SSF. The administration volume was standardized to 60 μL daily for all groups. During 60 days, the mice body weight and daily food and water consumption were recorded, along with their weekly weight. Subsequently, the OGTT was performed, and after 3 days of rest for the mice, the insulin tolerance test (ITT) was performed. At the conclusion of the testing period, mice were sacrificed by decapitation in accordance with the guidelines set forth by NOM [NOM-062-ZOO-1999], and intestinal samples were harvested.
Monitoring body weight and food intake
The body weight of the mice was assessed weekly, and the body weight differences (Δ) were analyzed during the 1st, 4th, and 8th weeks, corresponding to the beginning, middle, and end of the experimental period. The mice weight was measured using a digital scale (KD 160, TANITA,Tokyo, Japan). The weight of pellets consumed daily was obtained to assess each group’s meal intake. Caloric intake was estimated based on the grams of food consumed per day according to the provider’s caloric intake reported (Table S1 in Supplementary Materials).
Acute response evaluation (with preload and without preload)
Each group was divided equally before starting the OGTT to evaluate the acute response to solution administration. Mice were fasted for 4 h, and glucose was measured after 3.5 h. Preloads were administered to the VC, PC, and PDF groups, followed by an oral glucose load (2 g/kg body weight). Glucose levels (mg/dL) were recorded at specific intervals, and the area under the curve (AUC) was calculated using the trapezoidal rule to assess total glucose exposure [Andrikopoulos et al., 2008].
Insulin tolerance test
Insulin-sensitive cells can be observed to see how well insulin stimulates the internalization of circulating glucose using the approved approach known as ITT [Gelding et al., 1994]. Mice were fasted for 4 h and administered intraperitoneally with 0.070 IU/kg insulin. Glucose was subsequently monitored at the same times mentioned above for OGTT.
Serum metabolic hormone analysis
Serum samples were obtained by dividing each study group into two subgroups (n=4 each) to evaluate metabolic hormone levels under fasting (4 h) and postprandial conditions. Sacrifices were conducted over 4 days (one group per day), with animals maintained on a standard diet and without experimental solutions before assignment to fasting or postprandial subgroups. Blood was centrifuged to isolate serum, and metabolic hormone concentrations glucose-dependent insulinotropic polypeptide (GIP), insulin, leptin, and resistin were measured using the MILLIPLEX MAP Mouse Panel Kit (MMHE-44K, Millipore,Burlington, MA, USA).
Short-chain fatty acid analysis
After sacrifice, cecal contents (n=4) were carefully collected in microtubes and stored at −80°C. The quantification of SCFAs was performed using a Shimadzu GC 2010 Plus gas chromatograph equipped with a flame ionization detector (FID) (Shimadzu Scientific Instruments, Kyoto, Japan). Samples (20–35 mg) were homogenized in distilled water and subjected to a liquid-liquid extraction method using a solvent mixture consisting of n-butanol, tetrahydrofuran, acetonitrile, 0.1 M HCl, citric acid, and sodium chloride, following the protocol described by Ribeiro et al. [2018]. The supernatant was obtained by centrifugation at 13,000×g for 10 min and filtered using a Whatman GD/X syringe filter with 0.22 μm polyvinylidene fluoride (PVDF) membrane (MERCK, Billerica, MA, USA). Chromatographic analysis was performed using a high-polarity Mega-Acid column (MEGA, Legnano, Italy). Data were processed with LabSolutions software (Shimadzu), and the contents of SCFAs, specifically acetic acid, butyric acid, and propionic acid, were determined using 6-point standard curves (12.5, 25, 50, 100, 200, and 400 mg/L).
Goblet cell count
Descending colon tissue samples were embedded in paraffin and sectioned to 5 µm thickness. Each sample slide included five tissue sections, stained with Alcian blue (HYCEL, Zapopan, Mexico) and hematoxylin to identify goblet cells. Goblet cells were counted in three randomly chosen sections per sample, with 10 crypts assessed per section, totaling 30 crypts per sample. Using APERIO Image Scope software (Leica, Vista, CA, USA) at 20× magnification (100 µm), results were recorded as goblet cells per crypt per 100 µm [Rodríguez-Mejía et al., 2022].
Statistical analysis
Statistical analyses were conducted using GraphPad Prism software (version 8.0.1, San Diego, CA, USA). Descriptive statistics, including mean and standard deviation, were applied to the proximate chemical and phenolic compound analyses of PDF. These analyses were conducted in triplicate. For evaluations of data from in vivo experiment, one-way analysis of variance (ANOVA), two-way ANOVA or the non-parametric Kruskal-Wallis test was used, depending on the normality of the data (assessed with the Shapiro-Wilk test), to identify significant differences between groups (p<0.05). Post hoc comparisons were performed using Tukey’s test or Dunn’s test, as appropriate.
RESULTS AND DISCUSSION
Nutritional and phenolic content in P. dulce flour
The proximate composition of PDF, revealing a crude protein content of 9.26 g/100 g dm, is provided in Table 1. This protein content was lower than the 12–15 g/100 g dm reported for dehydrated P. dulce arils from India [Rao et al., 2011]. The levels of moisture, ash, total lipids, and total carbohydrates were like those reported in the cited study.The proximate composition of fresh P. dulce arils has been previously reported on a fresh matter (fm) basis, though the values varied due to methodological differences and environmental factors during plant harvest. For instance, in regions of Mexico such as Sinaloa, the arils exhibited a high moisture content (77.20 g/100 g fm), a low protein content (2.48 g/100 g fm), and significant levels of dietary fiber (5.97 g/100 g fm) [Pio-León et al., 2013].
Table 1
Nutrient composition, phenolic compound content, and antioxidant capacity of Pithecellobium dulce (Roxb.) Benth. fruit flour.
Proximate analysis of PDF (Table 1) revealed a total DF content of 10.48 g/100 g dm, with 7.77 g/100 g dm as insoluble fiber and 2.71 g/100 g dm as soluble fiber, primarily pectin. The predominance of insoluble fiber suggested the presence of hemicelluloses previously identified in P. dulce arils [Preethi & Mary Saral, 2016].
Like DF, phenolic compounds play a critical role in modulating glycemic response and are integral to the food matrix. P. dulce contains a variety of phytochemicals, including flavonoids, tannins, glycosides, saponins, phytosterols, triterpenes, and alkaloids [Pradeepa et al., 2013]. To evaluate the potential functional benefits of PDF, its total phenolic content, total flavonoid content, tannin content and antioxidant capacity were assessed (Table 1). The total phenolic content was notably high as consistent with previously reported values for dehydrated P. dulce arils, which range between 462.40 and 829.80 mg GAE/100 g depending on the aril color (white or red) [Rao et al., 2011].
Interestingly, the total phenolic content in fresh materials was not significantly different (392.2 and 517.8 mg GAE/100 g fm in white and red arils, respectively) [Pio-León et al., 2013]. While dried fruit flour might be expected to have higher phenolic content, dehydration can also cause a reduction in phenolic compounds. Compared to other commonly consumed fruits in their fresh state, such as raspberries (383–400 mg GAE/100 g fm) [Mihailović et al., 2019] and blueberries (275–645 mg GAE/100 g fm) [Hernández-Carrión & Caicedo Narvaez, 2022], the PDF contained a respectable amount of phenolics. The current study reports a higher total flavonoid content in the PDF compared to fresh arils, with 50.0 and 86.6 mg quercetin equivalents/100 g in white and red arils, respectively [Pio-León et al., 2013]. These levels were comparable to those in pomegranates (84–214 mg CE/100 g) [Shams Ardekani et al., 2011] and colored grapes (210.6–758.2 mg CE/100 g) [Liang et al., 2014], known for their high antioxidant capacity. Consistently, the antioxidant capacity of the PDF (656 µg TE/g dm) underscored the significant contribution of the bioactive compounds present in the sample. Additionally, tannin content in the PDF was lower than in fresh arils (148.2 and 309.2 mg/100 g in white and red arils, respectively) [Pio-León et al., 2013]. Tannins, common in many plants, are known for their astringency and antinutritional properties, and their content may be reduced during food processing steps like soaking [Ojo, 2022]. The preparation of the fruit before dehydration has likely contributed to the reduced tannin levels in the flour.
Dose standardization
Dose-response evaluation of P. dulce flour
Glycemic curves obtained to establish the effective dose of PDF are shown in Figure 1A. The highest glucose peak was observed at 15 min in all groups. The VC group showed a decrease in their glucose levels from the 30th minute with a slow reduction to 120 min. The group administered the 25.0 mg/kg dose showed higher glucose levels, observing a marked decrease up to the 60th minute. The dose group of 12.50 mg/kg showed a shortened and delayed curve over all measured times to reach their baseline values. The lowest dose group (6.25 mg/kg) showed a lower glucose concentration in the 15th minute (below 20 mg/dL), shortening the time to reach their baseline blood glucose values. When compared to doses of 25.00 mg/kg (p<0.0001), 12.50 mg/kg (p<0.001), and the VC group (p<0.05), the dose of 6.25 mg/kg resulted in lower glucose concentrations, according to the AUC (Figure 1B) which displays the glucose concentration present in the groups with different doses during the 120 min test.
Figure 1
Glucose dose-response evaluation of Pithecellobium dulce (Roxb.) Benth. fruit flour (PDF) at 6.25, 12.50, and 25.00 mg/kg. (A) Oral glucose tolerance test (OGTT). (B) Area under the curve (AUC). Statistical analysis: one-way analysis of variance and Tukey’s post hoc test. Significant differences: ***p<0.001, *p<0.05. VC, vehicle control.
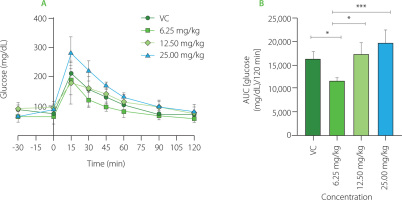
The sugar content in the dry matter of P. dulce fruit has been reported to vary depending on its ripeness. These sugars include fructose (12–14 g/100 g), glucose (11–14 g/100 g), and sucrose (2–4 g/100 g) [Wall-Medrano et al., 2016]; in addition, monosaccharides such as xylose, rhamnose, ribose, galactose and mannose have been extracted from the fruit [Preethi & Mary, 2016].
PDF has been obtained and developed from the whole fruit, which is why the establishment of the most effective dose was required to begin prolonged administration. As indicated in the dose-response evaluations, the lowest dose (6.15 mg/kg/day) demonstrated superior efficacy in reaching basal glucose levels by obtaining lower blood glucose levels throughout the OGTT. Findings demonstrated that PDF did not provide a larger reaction at higher dosages, which might happen if its mechanism of action to enhance glycemic control was caused by DF viscosity in the chyme, which functions in a dose-dependent manner, particularly in soluble fiber. DF properties are related to the cell wall matrix of its polysaccharides (porosity, cell rupture and viscosity), which can affect the bioaccessibility of nutrients, gastric emptying and gastrointestinal transit rates, as well as the degree of digestion and absorption of macronutrients [Giuntini et al., 2022]. DF is involved in nutrient encapsulation, remaining structurally intact in plant tissues, which slows down and impairs digestion, thus reducing postprandial glycemic increase [Holland et al., 2020]. However, it has been suggested that xylose could be a potent sucrase inhibitor, mitigating postprandial glucose increase [Bae et al., 2011]. In this context, xylose has been found able to immediately decrease postprandial peaks in blood glucose and insulin in healthy individuals after ingesting this monosaccharide together with sucrose. The xylose efficacy combined with a high-protein and high-fat food matrix (muffins) was also investigated by the authors. They observed a significant decrease in postprandial glycemia responses, however, not as much as when xylose was consumed alone with a sucrose solution. These findings imply that the glycemic response in OGTTs at an acute dosage observed in our study might have been influenced by the xylose present in PDF. In vitro digestion models have demonstrated the efficacy ofphenolic compounds found in certain fruits in inhibiting enzymes involved in the metabolism of carbohydrates, particularly in anthocyanin-rich fractions [Prpa et al., 2021].
In addition to DF, the white and red arils of P. dulce are rich in phytochemicals, including high levels of vitamin C and anthocyanins, especially in red arils [Pio-León et al., 2013]. In vitro, the methanolic extract from the red arils demonstrated superior α-glucosidase inhibition compared to the reference drug, acarbose. This effect is primarily due to anthocyanins such as cyanidin 3-O-glucoside and pelargonidin 3-O-glucoside, which interact with α-glucosidase in both competitive and non-competitive ways. This enzyme, located in the small intestine, plays a key role in carbohydrate breakdown and its inhibition may help delay or reduce postprandial blood glucose spikes [López-Angulo et al., 2018]. The observed dose-response effect in this study is likely linked to the high phenolic content in the PDF. Additionally, the glycemic response is often influenced by the viscosity of soluble DF, which delays gastric emptying and forms a physical barrier that reduces nutrient absorption in the small intestine [Alexander et al., 2019].
Dose-response evaluation of inulin
Inulin was used as a positive control due to its prebiotic properties and its widespread use as a dietary supplement to enhance insulin sensitivity and metabolic syndrome markers [Li et al., 2021]. As a non-digestible compound in the small intestine, it is fermented in the colon, selectively promoting the growth of beneficial bacteria that produce SCFAs [Nakajima et al., 2022]. This prebiotic effect plays a key role in metabolic regulation. Additionally, inulin has been associated with reduced postprandial glycemic and insulinemic responses, as it does not contribute to blood glucose upon ingestion [Lightowler et al., 2018].
In the present study, different doses of inulin were evaluated to identify the best glycemic response during the OGTT (Figure S1A in Supplementary Materials). The results showed that the glycemic concentration in AUC for the maximum dose (50 mg/kg) was considerably lower than for the lower doses, though it remained quite close to the glycemic concentration reported for the VC group (Figure S1B).
Changes in body weight and food intake
The mice in the four groups had comparable average weight during the first week of the trial (Figure 2A-C). During the first month, there were significant differences between the UC and PDF groups’ Δ body weight values, showing that the UC group had gained weight while the PDF group had lost weight. By the eighth week, there had been no discernible variations in weight among the four research groups.
Figure 2
Changes in body weight during the study period: (A) first week, (B) fourth week, (C) eighth week. (D) Food intake at weeks 1, 3, and 6. (E) Caloric intake across the same time points. Statistical analysis: non-parametric Kruskal-Wallis analysis of variance (ANOVA) and Dunn’s post hoc test (A, B, C); one-way ANOVA and Tukey’s post hoc test (D, E). Significant differences: **p<0.01. Groups: UC, unmanipulated control; VC, vehicle control; PC, positive control (inulin-treated); PDF, treated with Pithecellobium dulce (Roxb.) Benth. fruit flour.
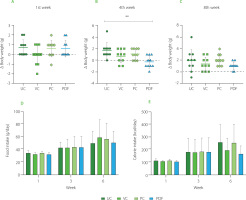
In the food intake and their respective caloric intake per week, no significant differences (p≥0.05) were observed between groups (Figure 2D and 2F). But there was a significant difference in food intake when comparing the first week to the sixth week of the study (p<0.001) across all groups, with a variation of 23.78%. Similarly, calorie intake showed a significant increase over time, with a notable difference between the first and sixth weeks (p<0.001), resulting in an 18.5% variation during this period. Animal studies on body weight and nutritional intake have demonstrated that eating different forms of fiber had a stronger impact on obesity [Isken et al., 2010]. In contrast, in the present study, mice did not have obesity, so the expected approach was to maintain body weight, as observed in all groups. Likewise, there were no differences in food intake between these. The addition of DF to diet may have an impact on the fluctuation in weight and dietary intake, even if the mice do not start the trial with altered weight. Mao et al. [2018] reported that large doses of fructo-oligosaccharides (FOS) (about 25% of diet) supplemented in non-obese mice resulted in dose-dependent body weight control. The animals also tended to reduce their food intake and exhibited soft, sticky feces. These effects have been related to intestinal microbiota modification promoted by the FOS prebiotic effect. Maintenance weight observed in all groups, including the PC, to whom inulin was administered, shows an effect like that reported by Zhang et al. [2018] regarding their control group made up of healthy rats administered with inulin (3 g/kg), after 12 weeks, the weight remained the same as in the group that was not administered with this DF.
Oral glucose tolerance test after 60 days of administration
Acute preloading of the solutions was done during OGTT at the end of the prolonged administration (Figure 3A and 3B). The AUC showed that the PDF group’s glucose levels were lower than the VC group’s (p<0.05). In the preload-free curves, the PDF group had lower glucose levels than the VC group (p<0.0001) and the inulin-administered group (p<0.0001) (Figure 3C and 3D). To determine the effects of the solution given before a high glucose load, the differences were evaluated between each experimental group’s performance on the OGTT with and without preloading solutions. AUC glucose did not change substantially between the VC and PC groups, but when a preload was not given, the level in the PDF groups was significantly lower (p<0.001) (244.60 mg/dL/15 min) vs. with preload (305.20 mg/dL/15 min) (Figure 3A and 3C, respectively).
Figure 3
Oral glucose tolerance test (OGTT) results after 60 days of administration. (A) OGTT in preloading groups. (B) Area under the curve (AUC) of preloading groups. (C) OGTT in non-preloading groups. (D) AUC of non-preloading groups. Statistical analysis: one-way analysis of variance and Tukey’s post hoc test. Significant differences: ****p<0.0001, *p<0.05. Groups: VC, vehicle control; PC, positive control (inulin-treated); PDF, treated with Pithecellobium dulce (Roxb.) Benth. fruit flour.
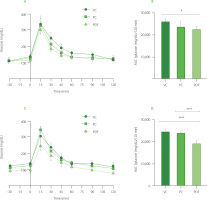
These results indicate that administering PDF for a prolonged period presented greater benefits in glycemic control after high sugar ingestion. According to these results, a meta-analysis has been reported on the benefits of glycemic control of chronic treatment with DF in patients with type 2 diabetes mellitus (T2DM), it reports a decrease in blood sugar, serum insulin, and fasting glycosylated hemoglobin [Mao et al., 2021]. However, the evidence regarding the type of fiber’s effectiveness in enhancing postprandial insulinemia and glucose response is inconsistent [Tsitsou et al., 2023].
The antidiabetic potential of P. dulce fruit is understudied, with existing research highlighting the hypoglycemic effects of its ethanolic aril extracts. In diabetic rats, 30 days of treatment (300 mg/kg/day) improved glucose tolerance and reduced blood glucose, glycated hemoglobin, urea, and creatinine levels [Pradeepa et al., 2013]. These effects were linked to inhibited glucose absorption, enhanced glycogen storage, and increased insulin secretion. In contrast, in this study, flour derived from dried P. dulce arils was used, obtained through dehydration without the addition or removal of any components. The resulting PDF primarily contains carbohydrates (57.44 g/100 g dm), dietary fiber (10.48 g/100 g dm), and protein (9.26 g/100 g dm), while also containing a notable amount of phenolics (Table 1).
The effects of this flour on mice may depend on the various compounds it contains. The metabolic state of the rodents plays a crucial role in how DF affects glycemic regulation. A meta-analysis found that FOS supplementation reduced fasting glycemia more significantly in conditions like obesity, T2DM, or a high-fat diet compared to healthy animals [Le Bourgot et al., 2018]. In this study, despite using healthy mice, prolonged PDF consumption improved glycemic regulation, suggesting that these effects could be stronger under impaired glucose homeostasis. The response to the OGTT in the PC group was like that of the VC group, highlighting that inulin’s beneficial effects on glucose regulation are most evident in altered metabolic states. Zhang et al. [2018] observed that inulin supplementation in diabetic rats enhanced the abundance of beneficial SCFAs-producing bacteria. However, in healthy rats, inulin had no effect on the diversity of the intestinal microbiota.
Furthermore, the greater effects observed in the PDF group compared to the PC group are attributed to the integrity of the food matrix in the PDF, which in addition to containing fermentable fiber fractions, was a rich source of phenolics. In contrast, the chicory inulin used in the PC group is a purified source. These results contrast with the findings reported by Fotschki et al. [2019], who compared the effects of chicory root flour and pure FOS in rats with necrotic colitis. In their study, greater benefits were found with the use of chicory root flour, due to its greater complexity in terms of fibers and bioactive compounds, which were associated with an increase in SCFAs production and improved intestinal integrity regeneration. These results highlight the importance of using whole dietary ingredients rather than purified supplements to regulate the intestinal microbiota and improve inflammatory conditions.
Insulin tolerance test
The ITT curves were performed during the last week of prolonged solution administration. As shown in Figure 4A, both the PC and PDF groups exhibited a progressive decrease in glucose at 30 min, while the VC group continued to decrease until 45 min. All three groups showed variable glycemic responses until their basal levels were restored at 120 min. In terms of the AUC (Figure 4B), no significant differences (p≥0.05) in glucose concentrations were observed. The ITT reflects insulin action by measuring the extent of glucose reduction after insulin administration. Since the half-life of insulin in mice is approximately 10 min, glucose reduction after 30 min may not be a direct effect of insulin, but rather a counterregulatory response involving hormones such as glucagon, catecholamines, and cortisol when glucose levels fall below 80 mg/dL [Ayala et al., 2010]. Under normal conditions, increased blood glucose stimulates insulin secretion, which promotes glucose uptake by peripheral tissues and inhibits hepatic gluconeogenesis [Li et al., 2022]. Despite differences in glycemic regulation observed in the PDF group, the homeostatic response to insulin was similar across all groups, likely due to the BALB/c strain’s metabolic phenotype. This strain has been shown to maintain glucose tolerance and insulin sensitivity even under high-fat diet conditions [Benedé-Ubieto et al., 2020].
Figure 4
(A) Insulin tolerance test (ITT) results after 60 days of administration. (B) Area under the curve (AUC) of glucose concentrations during the 120-min ITT. Statistical analysis: one-way analysis of variance and Tukey’s post hoc test. No significant differences (p≥0.05) in AUC between groups. Groups (n=5): VC, vehicle control; PC, positive control (inulin-treated); PDF, treated with Pithecellobium dulce (Roxb.) Benth. fruit flour.
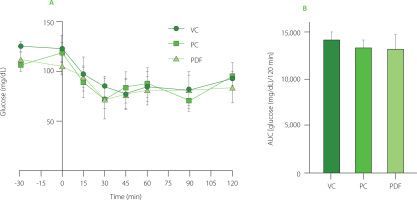
Serum concentration of metabolic hormones
To determine the concentration of hormones that play an important role in glucose metabolism, serum samples were analyzed under fasting and postprandial conditions. In fasting, no significant differences (p≥0.05) were reported between the PDF group and the other groups (Figure 5A). GIP levels in the PDF group were lower in the postprandial state compared to the other groups, although the differences did not reach statistical significance. This trend suggests that P. dulce may attenuate the GIP response following food intake. This effect could be associated with the presence of phenolics and fermentable fiber in the fruit, which modulate hormonal responses through mechanisms such as delayed gastric emptying and interaction with the gut microbiota, impacting the release of incretins like GIP [Guccio et al., 2022; Williamson, 2022]. However, a larger sample size is required to confirm these findings.
Figure 5
Serum concentrations of metabolic hormones: (A) Glucose-dependent insulinotropic polypeptide, GIP, (B) insulin, (C) leptin, (D) resistin. Groups (n=4) evaluated under fasting and postprandial conditions. Statistical analysis: one-way analysis of variance (ANOVA) and Tukey’s post hoc test; resistin was analyzed using Kruskal-Wallis ANOVA and Dunn’s post hoc test. Significant differences: **p<0.01, *p<0.05. Groups: UC, unmanipulated control; VC, vehicle control; PC, positive control (inulin-treated); PDF, treated with Pithecellobium dulce (Roxb.) Benth. fruit flour.
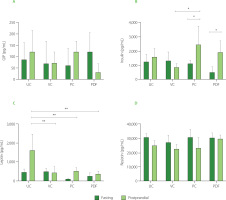
The insulin response in the postprandial state was significantly higher (p<0.05) compared to fasting conditions in the PC and PDF groups (Figure 5B), indicating greater insulin sensitivity. This effect was not observed in the control groups (VC and UC). As the solutions were not administered during the three days preceding sacrifice (in the PC and PDF groups), the serum samples collected for metabolic hormone analysis reflect cumulative effects from prolonged administration rather than acute responses to the solutions. These cumulative effects were likely attributable to the fiber and phenolics within the PDF matrix. The phenolic compounds present in PDF may have accumulated in tissues, exerting sustained antioxidant and anti-inflammatory effects [Yahfoufi et al., 2018]. Furthermore, phenolic compounds can influence the modulation of the expression of genes related to glucose metabolism, improving insulin sensitivity [Kang et al., 2020]. It is possible that, despite the discontinuation of PDF consumption for four days, these compounds continue to have a modulatory effect on the metabolic pathways that regulate insulin and GIP levels.
Anthocyanins have demonstrated positive effects on glucose metabolism, including the neutralization of oxidative stress, prevention of apoptosis in pancreatic β cells, and regulation of genes involved in glucose transport, insulin sensitivity, and hepatic glucose production [Kang et al., 2020]. Studies on mice and human 3T3-L1 adipocytes have shown that anthocyanins, such as cyanidin-3-O-glucoside, and their metabolites, such as protocatechuic acid, improve insulin sensitivity and promote glucose uptake by facilitating the translocation of GLUT-4 to the cell membrane. Furthermore, they positively regulate PPAR-γ and the adiponectin gene [Scazzocchio et al., 2011]. PPAR-γ controls the expression of key proteins involved in glucose and fatty acid uptake, while adiponectin, produced by adipocytes, enhances insulin sensitivity. Together, these mechanisms play crucial roles in glucose and lipid metabolism [Den Besten et al., 2015].
Regarding leptin levels, only the control group (UC) showed significantly higher (p<0.01) serum concentrations in postprandial conditions (Figure 5C), consistent with the body weight fluctuations observed in this group (Figure 2C). Leptin levels are influenced by adipose tissue reserves and food availability. In the postprandial state, gastric leptin secretion responds to food intake and is modulated by energy reserves [Mendoza-Herrera et al., 2021]. The UC group’s characteristics may reflect stress from minimal experimental manipulation, causing maladjustment to standard conditions [Marin et al., 2023]. Additionally, stress in mice can induce leptin resistance, leading to uncontrolled eating and weight gain [Patterson & Abizaid, 2013]. The reduced serum leptin levels in the postprandial condition observed in the PC and PDF groups are consistent with the findings of Den Besten et al. [2015] who also reported a decrease in serum leptin levels in animals directly treated with SCFAs. This reduction was attributed to the lower white adipose tissue mass in these mice. Despite the lower levels, which indicate reduced satiety signaling, food intake in SCFAs-treated animals did not increase, a pattern that aligns with the feeding behavior observed in the present study.
Serum resistin concentrations represent a relevant marker to evaluate the health status of the mice in the study, since the levels recorded in all groups remained within the physiological range of 40 to 80 ng/mL [Rajala et al., 2003], without significant differences (p≥0.05) between them (Figure 5D). In murine models, resistin has been shown to function as an antagonist in insulin signaling, and its elevated levels are implicated in the development of insulin resistance [Steppan et al., 2001]. Although the relevance of this relationship in humans remains a matter of debate, a recent meta-analysis indicated that serum resistin concentrations significantly correlate with insulin resistance in the contexts of obesity and T2DM [Su et al., 2019].
Changes in gut microbial metabolites
Administration of PDF significantly altered SCFAs production, underscoring its prebiotic potential. Analysis of cecal contents revealed a notable increase in propionic acid levels (p<0.05) and a trend toward higher acetic acid levels in the PDF group compared to the unmanipulated control (Figure 6A and 6B). Furthermore, butyric acid levels were significantly elevated in the PDF group compared to the UC group (p<0.01).
Figure 5
Short-chain fatty acid (SCFA) content in the cecum: (A) propionic acid, (B) acetic acid, (C) butyric acid. (D) total SCFA content by group. Statistical analysis: Kruskal-Wallis analysis of variance (ANOVA) and Dunn’s post hoc test (A–C); one-way ANOVA and Tukey’s post hoc test (D). Significant differences: **p<0.01, *p<0.05. Groups (n=4): UC, unmanipulated control; VC, vehicle control; PC, positive control (inulin-treated); PDF, treated with Pithecellobium dulce (Roxb.) Benth. fruit flour.
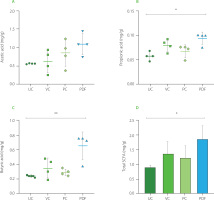
Fermentation occurs in the colon, particularly in regions with lower pH, such as the cecum, leading to higher SCFA contents. In the colon and stool, the typical molar ratio of acetic acid to, propionic acid, and butyric acid is 3:1:1, depending on microbiota composition, diet, and intestinal transit time [Nogal et al., 2021].
Overall, total SCFA contents were significantly higher in the PDF group, showing notable differences compared to the UC group (p<0.05) (Figures 6D). This suggests enhanced fermentation of dietary fibers in PDF, likely driven by its composition rich in fermentable polysaccharides such as hemicelluloses [Preethi & Mary, 2016].
The increase in acetic acid in the intestine reflects the activity of bacteria such as Bifidobacterium spp., with this SCFA acting as a functional mediator in various systemic metabolic processes. Its increased availability in the body has been linked to improved insulin sensitivity through the activation of the FFAR2 receptor on pancreatic β-cells. Furthermore, acetic acid exerts an anti-inflammatory effect by inhibiting pro-inflammatory cytokines and enhancing the function of the intestinal barrier, thereby supporting metabolic homeostasis and protecting against inflammatory disorders [Fusco et al., 2023].
The increase in propionic acid, associated with Bacteroidetes, further supports the observed metabolic benefits, as this SCFAs serves as a substrate for hepatic gluconeogenesis and stimulates enteroendocrine L cells to secrete GLP-1 and PYY, hormones essential for glucose regulation and appetite control [Psichas et al., 2015].
Butyric acid was the metabolite that stood out with significantly higher concentrations compared to the UC group, suggesting modifications in the microbiota and improvements in intestinal integrity. Given that butyric acid is a key energy source for colonocytes and a modulator of epithelial inflammation, it plays a crucial role in maintaining intestinal integrity and promoting glucose homeostasis through FFAR3-mediated gluconeogenesis. This SCFA is primarily produced by Faecalibacterium prausnitzii and Ruminococcus spp. [Salvi & Cowles, 2021], whose activity may have been stimulated by the fermentable fractions of PDF.
These findings underscore the potential of PDF to modulate gut microbiota and enhance SCFAs production, offering promising health benefits, particularly in metabolic regulation. However, future research employing microbiome sequencing is crucial to precisely identify the bacterial shifts underlying these effects.
Goblet cells count in descending colon
PDF significantly increased goblet cells per crypt in the descending colon (Figure 7), suggesting a possible improvement in the epithelial barrier. This finding is consistent with that reported by Yang et al. [2024] who demonstrated that fermentable fibers such as arabinoxylan and resistant starch increase both the number of goblet cells and the thickness of the mucosa, favoring the integrity of the intestinal barrier. Similarly, the inulin-treated group (PC) showed a significant increase in these cells compared to UC and VC (Figure 7A). This aligns with the results reported by Corrêa et al. [2023] who associated inulin intake with remodeling of the colonic epithelium, promoting the proliferation and differentiation of intestinal stem cells, as well as the improvement of the intestinal barrier and mucin secretion.
Figure 5
(A) Goblet cells count in the ascending colon. Histological staining with Alcian blue and hematoxylin in (B) unmanipulated control (UC), (C) vehicle control (VC), (D) positive control (PC), and (E) Pithecellobium dulce (Roxb.) Benth. fruit flour (PDF) groups (n=4). Statistical analysis: two-way analysis of variance and Tukey’s post hoc test. Significant differences: ****p<0.0001, *p<0.05.
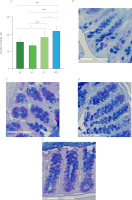
The intestinal mucosal barrier is essential for preventing pathogen translocation, with goblet cells, which secrete mucus containing antimicrobial peptides, playing a key role in microbiota regulation and intestinal transit [Gustafsson & Johansson, 2022]. In this study, mice treated with PDF exhibited a significant increase in the number of goblet cells in the colonic crypts, possibly associated with the fermentation of dietary fibers and the production of metabolites such as butyric acid, which was found at higher concentrations in this group. These findings are consistent with previous studies highlighting the role of fermentable fiber as an energy substrate for colonocytes, promoting intestinal homeostasis [Fusco et al., 2023]. Similarly, Hunt et al. [2021] reported that fiber consumption over 112 days in healthy mice increased intestinal weight, stimulated epithelial cell proliferation, and maintained intestinal integrity, in contrast to mice fed a fiber-deficient diet, underscoring its preventive potential in supporting intestinal health.
While promising, these results do not establish a direct link between PDF consumption and improved intestinal barrier integrity, as functional markers of barrier function were not assessed. Additionally, the absence of a taxonomic analysis of the microbiome limits the ability to pinpoint specific changes in its composition that might explain these effects. Nevertheless, the observed increase in SCFAs in the PDF group may be associated with certain bacterial phyla involved in fiber fermentation, hinting at potential interactions between PDF fiber and the intestinal microbiota.
Overall, the effects observed after prolonged PDF administration in healthy mice highlight the potential of its bioactive compounds to modulate SCFA production and potentially influence metabolic processes associated with intestinal health. However, these findings should be interpreted cautiously and serve as a foundation for further research to elucidate the underlying mechanisms and evaluate relevant functional markers.
The extrapolation of these results to humans is complex due to interindividual variability in the microbiota, influenced by factors such as diet, lifestyle, genetics, and medications [Nogal et al., 2021]. Although SCFAs, such as butyrate and propionate, have shown benefits in preclinical models [De Vadder et al., 2014; Psichas et al., 2015], the evidence in humans is limited and sometimes contradictory [Shortt et al., 2018]. Their effects, such as improving insulin sensitivity and intestinal integrity, largely depend on the interaction between diet, microbiota, and metabolic status [Ahrén, 2022]. Therefore, clinical trials are crucial to establish optimal dosages and assess their efficacy in humans, considering metabolic and digestive differences between species. These complexities underscore the need for broader research to understand the translational potential of PDF in human health.
CONCLUSIONS
This study highlights the preliminary functional potential of PDF as a dietary intervention to support glucose homeostasis, opening the door to future research on metabolic disorders. The observed metabolic effects suggest the need for further exploration of the pathophysiological mechanisms involved in glucose dysregulation, such as those seen in obesity, T2DM, and metabolic syndrome. However, the translational application of these findings to humans is complex due to the physiological and metabolic differences between murine models and humans. This underscores the need for more comprehensive research to adequately evaluate the potential of PDF in human health. By addressing these gaps, it may be possible to integrate P. dulce fruit consumption into clinical practice and develop functional products derived from this promising food source, but this requires further studies to validate its clinical applicability.