INTRODUCTION
Tea plant (Camellia sinensis (L.) O. Kuntze) originates from China. Nowadays, the infusion of tea leaves is one of the most popular beverages in the world. It is made of leaves subjected to a different degree and nature of fermentation [Wong et al., 2022; Sun et al., 2022]. Nevertheless, green tea, i.e. fresh leaves treated only with steam, rolled and dried, is also a very popular commercial product. Production of green tea accounts for 20% of the total tea production [Sun et al., 2022]. During gentle leaf processing to obtain green tea, oxidative enzymes are inactivated and no fermentation occurs; therefore, the major compounds of the leaves remain in their native form [Wong et al., 2022]. The dominant compounds of leaves and green teas are flavan-3-ols, including (−)-epigallocatechin gallate, (−)-epigallocatechin, (−)-epicatechin gallate, (−)-epicatechin, (−)-gallocatechin gallate, and (+)-catechin [Jiang et al. 2019]; followed by (+)-gallocatechin and (−)-catechin gallate [Svoboda et al., 2015]. The flavan-3-ol content can be as high as 125.4 mg/g dry weight [Svoboda et al., 2015]. Phenolic acids, flavonols, flavones, flavanonols, proanthocyanidins and derivatives of compounds of each of these phenolic classes were also identified among green tea phenolics [Janiak & Amarowicz, 2018; Scoparo et al., 2012; Shi et al., 2022]. Moreover, green tea is a rich source of purine alkaloids with caffeine as a major compound [Jiang et al., 2019; Scoparo et al., 2012]. Green tea phenolics, especially flavan-3-ols are well known for their antioxidant activity [Carloni et al., 2013; Peluso & Serafini, 2017]. They are also responsible for a wide range of other bioactivities including anti-inflammatory, antidiabetic and anticancer activities, antiobesity and immunoregulatory effects, as well as cardiovascular- and hepato-protective properties [Tang et al., 2019]. Therefore, there is a growing interest in not only infusions but also green tea extracts as a health-promoting component of the diet [Bártíková et al., 2017; Tang et al., 2019]. Green tea extracts are also increasingly applied, as a natural alternative to chemical antioxidants, in food technology to extend the shelf life of food products [Senanayake, 2013]. Their effectiveness in lipid oxidation inhibition in meat products has been widely confirmed [Oswell et al., 2018; Shah et al., 2014]. To this end, antioxidants have been extracted from green tea using different methods [Vuong et al., 2011], including solvent extraction with water or organic solvents as the most popular one.
From a nutritional point of view, green tea can be considered a valuable source of elements. It is rich in macroelements including calcium (Ca), potassium (K), magnesium (Mg), sodium (Na), and phosphorus (P) [Brzezicha-Cirocka et al., 2016; Koch et al., 2018]. Among essential trace elements, iron (Fe), copper (Cu), zinc (Zn), nickel (Ni), manganese (Mn), cobalt (Co), molybdenum (Mo), selenium (Se), and chromium (Cr) have been detected with the highest contents of Mn and Fe [Barone et al., 2016; Koch et al., 2018; Ma et al., 2019]. The transfer of individual elements from green tea to infusion has been shown to vary; however, Fe was less released from tea matrix to hot water than other trace elements [Koch et al., 2018; Wróbel et al., 2000]. Nevertheless, due to the high intake of tea beverages, it significantly contributes to meeting the daily dietary requirements of essential elements [Brzezicha-Cirocka et al., 2016; Chowaniak et al., 2021]. Although the trace element profile of green teas and their infusions has been studied depending on many variables, such as the geographical origin of the plants, their cultivar and growing conditions as well as leaf processing and infusion practices [Brzezicha-Cirocka et al., 2016; Deka el al., 2021; Na Nagara et al., 2022], the knowledge of the content of trace elements in the extracts and transfer of these elements to extracts is limited.
Elements, which are transition metals can bind to polyphenols forming stable complexes [Karamać & Pegg, 2009; Kejík et al., 2021; Samsonowicz & Regulska, 2017]. Considering essential trace elements of green tea, the complexation of iron, zinc, cooper, manganese, and chromium ions by flavan-3-ols has been demonstrated so far [Bronco et al., 2005; Cherrak et al., 2016; Lee & Heffern, 2022; Navarro et al., 2005]. Our previous study also showed the formation of complexes between ferrous ions and phenolic compounds of the high molecular weight fraction isolated from a green tea extract [Janiak & Amarowicz, 2018]. It has been suggested that interactions of polyphenols with metal ions may affect the transfer of these metals from green tea to infusions [Szymczycha-Madeja et al., 2012]. It seems that complexation may be even more important for the transfer and content of metals in green tea extracts, where phenolic compounds are concentrated. Therefore, the aim of our research was to determine the contents of selected essential trace elements, i.e., Fe, Zn, Cu, Mn, and Cr, in commercially available green teas and their extracts to evaluate the transfer of these elements from teas to extracts. Moreover, our aim was to verify that the major bioactive compounds of the extracts affect this transfer.
MATERIALS AND METHODS
Materials and chemicals
Twelve green teas were purchased from local stores in Olsztyn, Poland. Tea No. 1 was bagged and the rest of the teas were bought in the form of tea leaves. Other characteristics of the green teas used are present in Table 1. The chemicals used for extraction and the standards: caffeine, (–)-epigallocatechin (EGC), (–)-epicatechin (EC), (–)-epigallocatechin gallate (EGCG), and (–)-epicatechin gallate (ECG), were purchased from Sigma-Aldrich Chemical Co. (St. Louis, MO, USA). Nitric acid and ICP multielement standard solution IV CertiPUR (1000 mg/mL in nitric acid) were purchased from Merck (Darmstadt, Germany). The reference material – tobacco leaves ORIENTAL (CTA-OTL-1) – was supplied by the Institute of Nuclear Chemistry and Technology, Warszawa, Poland. Deionised water was obtained by the NANOpure Diamond water purification system (Barnstead, Dubuque, IA, USA).
Table 1
Characteristics of green teas used as research material and yield of their extraction with 80% (v/v) acetone.
Extraction
Green teas were powdered using a mortar and a pestle. Approximately 20 g of such prepared powders were extracted with 80% (v/v) acetone at a solid to solvent ratio of 1:10 (w/v) for 15 min at 50 C using the water bath JULABO SW22 (JULABO GmbH, Seelbach, Germany) [Slavova-Kazakova et al., 2021]. The liquids were filtered by Whatman No. 3 paper, and the residues were suspended in the next portions of solvent and re-extracted. The operation was done three times. Then, filtrates were combined. Organic solvent was removed using a rotary evaporator (R-210, Büchi Labortechnik AG, Flawil, Switzerland) and any remaining water residue was frozen and lyophilised (FreeZone 6 Liter Console System, Labconco, Kansas City, MO USA). The dried extracts were ground to a powder in a mortar and stored in tightly closed vials until analyses.
Mineralisation
Green teas and their extracts dried to a constant mass were placed in Teflon vessels. Portions of 0.16–0.29 g of green teas or extracts and 0.2841 g of tobacco leaves were weighed. Next, 6 mL of nitric acid were added and vials were sealed. The process of cold mineralisation spanned for 16 h. Afterwards, the material was degassed and transferred to a microwaveassisted mineraliser (MARS 5X, CEM Corporation, Matthews, NC, USA). Mineralisation (1200 W) was conducted for 57 min in constant pressure mode (max 55.19 bar). From 0–4 min, the temperature was raised to 160°C and maintained for 4 min, at 8–12 min it was raised to 180°C and maintained for 4 min, at 16–20 min it was raised to 200°C and kept for 7 min. Afterwards, the system was cooled for 30 min to restore initial conditions. The processed samples were degassed, diluted in deionised water, and transferred to 25-mL volumetric flasks. The same procedure was carried out for reference material and blank. Blank was prepared without sample addition. Thus obtained material was kept at 4°C until further use.
Element analysis
Mineralised green teas and extracts were analysed by inductively coupled plasma optical emission spectroscopy (ICP OES) using an iCAP 6000 series ICP-OES spectrometer coupled with a charge injection device (Thermo Electron, Waltham, MA, USA). Analysis was carried out in the plasma axial viewing mode with radio frequency generator set up to 1150 W. Samples were introduced to the system with a flow rate of nebulizer gas set to 0.5 L/min. Cu, Fe, Zn, Mn, and Cr were identified in the samples using spectral line wavelengths: 324.754 nm, 259.940 nm, 213.856 nm, 257.610 nm, and 284.325 nm, respectively. Samples were analysed in 3 repetitions. Calibration curves were prepared for each element from a stock standard solution (10 μg/mL) and appropriate dilutions (0.01, 0.2, 0.5, 1.0, and 2.0 μg/mL) to evaluate the content of metal ions in the samples, which was expressed in μg per g of green tea or extract. Additionally, the transfer (TR, %) of individual green tea elements to extract was calculated based on extraction yield.
Analysis of the contents of caffeine and flavan-3-ols using high performance liquid chromatography in reverse phase (RP-HPLC)
Green tea extracts were dissolved in the mixture of acetonitrile, water, and trifluoroacetic acid (5:95:0.1, v/v/v) (2 mg/mL) and filtered (0.22 μm, polyethersulfone membrane filter, TPP Techno Plastic Products AG, Trasadingen, Switzerland). Next, they were analysed after injection of 20 μL of each sample into the Luna C18 column (250×4.6 mm, 5 μm, Phenomenex, Torrance, St. Louis, MO, USA) attached to the chromatographic system consisting of two LC-10AD pumps, an SCL10A controller, a CTO-10AS column oven, and an SPD-M 10A detector (Shimadzu, Kyoto, Japan). Elution was conducted under binary gradient conditions [Janiak et al., 2017; Karamać et al., 2020]. The mobile phase consisted of two mixtures: A – acetonitrile:water:trifluoroacetic acid (5:95:0.1, v/v/v) and B – acetonitrile:trifluoroacetic acid (100:0.1, v/v). Gradient was set up as 0–40% B in 0–50 min. Flow rate was 1 mL/min. Peaks were recorded at 280 nm and their retention times were compared to those obtained for standards: EGC, caffeine, EC, EGCG and ECG. Calibration curves were prepared for each standard for quantification of compounds in extracts and green teas. Results were expressed as mg per g tea/extract.
Statistical analysis
All analyses were performed in triplicate. Data are presented as means ± standard deviations. Principal component analysis (PCA) allowed examining the relationships between element contents in green teas and in extracts, and their transfer to extracts. Content and transfer of each element were correlated with contents of the compounds detected by HPLC using Pearson’s correlation. All those analysis were performed using STATISTICA 10 (StatSoft Inc., Tulsa, OK, UAS).
RESULTS AND DISCUSSION
The contents of Fe, Zn, Cu, Mn, and Cr in green teas and their extracts as well as percentage of these elements transferred from teas to extracts are shown in Table 2. Among the analysed elements, in both green teas and extracts, high contents of manganese (711–1402 and 5.08–30.2 μg/g, respectively) and iron (115–725 and 10.7–90.1 μg/g, respectively) and the low content of chromium (0.509–1.75 and 0.128–2.03 μg/g, respectively) were determined. The level of copper was estimated in the range of 11.7–18.6 μg/g green tea and 1.93–13.8 μg/g extract. Contents of zinc in teas and extracts were 19.3–31.2 and 5.03–12.6 μg/g, respectively. Our findings for green teas are in accordance with results obtained by other researchers [Brzezicha-Cirocka et al., 2016; Koch et al., 2018; Na Nagara et al., 2022]. For instance, Koch et al. [2018] analysed green teas originating from different countries (China, India, Japan, Kenia, and Sri Lanka) and reported contents of 15.4–33.6 mg/100 g for Fe, 3.11–4.07 mg/100 g for Zn, 1.34– –2.03 mg/100 g for Cu, 39.0–126.0 mg/100 g for Mn, and 0.10–0.16 mg/100 g for Cr. However, other values were also noted in the literature, e.g., a lower level of Fe (54.14– –99.65 mg/kg) and a greater variation in the content of Cr (1.26–10.48 mg/kg) in green teas of different Indian cultivars [Deka et al., 2021]. Barone et al. [2016] determined a lower copper content (1.58–4.89 μg/g dry weight) and similar as in our study iron, chromium and zinc contents in green teas commercially available in Italy. In turn, Ma et al. [2019] reported much higher level of Zn (51.2–95.9 mg/kg) in Chine green teas, although the Mn, Cu, and Cr contents were within the ranges of those in our study. These differences may be due not only to the geographic origin and cultivar variation of the teas, but also to the plant growing conditions and method of post-harvest tea leaf processing [Brzezicha-Cirocka et al., 2016; Deka el al., 2021; Szymczycha-Madeja et al., 2012].
Table 2
Content of Fe, Zn, Cu, Mn, and Cr in green teas (GT) and their extracts (EX), and transfer of green tea elements to the extracts (TR).
The transfer of green tea elements to extracts varied over a wide range of 6.23–70.8% for chromium (Table 2). The transfer ranges for iron and copper were also wide, 1.33–25.4% and 3.46–27.8%, respectively, but in these cases the result for one sample significantly affected the scattering; excluding tea No. 1 for Fe and tea No. 11 for Cu, the transfer ranges were 1.33–9.14% and 11.4–27.8%, respectively. The values for zinc and manganese were the least differentiated, 7.26–15.8% and 0.269–0.646%, respectively. Thus, the lowest transfer of green tea manganese to extract was found with the mean value of 0.463%. The low value for iron (mean, 5.52%) can also be highlighted. Correlations between extraction yield and percentage of individual green tea elements transferred to the extract were assessed, and a significant negative correlation (correlation coefficient, r=–0.8018) was found only in the case of manganese. To the best of our knowledge, there is a lack of studies regarding the transfer of elements from green teas to their extracts. However, many authors have investigated the transfer of tea elements to infusions [Barman et al., 2020; Dambiec et al. 2013; Schulzki et al., 2017; Wróbel et al., 2000; Zhang et al., 2018]. Szymczycha-Madeja et al. [2012] grouped the elements taking into account the efficiency of their extraction from various types of teas to infusions, and classified iron as “poorly extractable” (<20%), while zinc, copper, manganese and chromium as “moderately extractable” (20–55%) group. In the case of green teas, the percentage of elements transferred to hot water during the preparation of beverages was 5.36–8.1% for Fe, 28.1–36.9% for Zn, 22.4–54.0% for Cu, 23.7–44.6% for Mn, and 25.0–45.4% for Cr [Koch et al., 2018; Schulzki et al., 2017; Wróbel et al., 2000; Yang et al., 2022]. Different values were noted by Chowaniak et al. [2021] who analysed the elements of green teas from Chinese cultivated and wild plants and their infusions: 11.5 and 11.9% for Cu, 11.1 and 11.0% for Fe, 9.9 and 9.8% for Mn, and 10.6 and 11.6% for Zn. Taking into account that the mean transfer of individual elements in our study accounted for 5.52% (Fe), 10.4% (Zn), 20.0% (Cu), 0.463% (Mn) and 26.2% (Cr), it can be stated that iron, copper, and chromium were extracted with 80% (v/v) acetone at levels similar to those obtained with hot water. The transfer of zinc and manganese to the extracts could be classified as poor according to the classification for infusions. A particularly large difference for manganese was evident in this comparison. The transfer of elements to infusions depends on the strength of their bonding to the tea matrix compounds and their hot water solubility [Szymczycha-Madeja et al., 2012]. It can be assumed that some manganese species were released from the tea matrix with 80% (v/v) acetone to the lesser extent than with water. Besides, due to a high Mn content in green tea (Table 2), its solubility in a solvent containing only 20% (v/v) of water could be hampered. Another reason can be found in the weak binding of Mn with the extracted compounds. Aqueous acetone was selected as an extractant in our study to maximise the extraction of green tea phenolic compounds. Perva-Uzunalic et al. [2006] reported that the use of hot aqueous acetone allowed obtaining a higher extraction efficiency of flavan-3-ols from green tea than aqueous ethanol, aqueous methanol or hot water.
The results of principal component analysis (PCA), that was conducted to highlight the differences in the content of elements in green teas and extracts as well as transfer of those elements to the extracts, are shown in Figure 1. The first two principal components (PC1 and PC2) explained most of the total variance (65.35%). Whereas, four principal components (PC1–PC4) described 88.43% of the total variance. Figure 1A presents variables that differentiated distribution of the samples. Variables were positively or negatively correlated with PC1, which is visible in the two separate groups on the plot; positively correlated variables are shown on the right side and negatively correlated variables are grouped on the left side. In the case of Cu, Fe, and Cr contents in green teas, correlations were opposite to correlations of these element contents in the extracts and their transfer to extracts. Zn content and transfer to the extract correlated oppositely to the analogous variables for Mn. PC2 was correlated with variables mostly positively, which was indicated by their clustering in the upper part of the PCA plot. Cr and Fe contents in green teas, Mn content in green teas and extracts as well as transfer of Mn to the extract were strongly positively correlated with this component.
Figure 1
Principal component analysis (PCA) plots of distribution of variables (A for PC1 and PC2; C for PC3 and PC4) and distribution of observations (B for PC1 and PC2; D for PC3 and PC4). Numbers 1–12 correspond to the numbers of the tea samples. CuGT, FeGT, ZnGT, MnGT, and CrGT – contents of Cu, Fe, Zn, Mn, and Cr in green teas, respectively; CuEx, FeEx, ZnEx, MnEX, and CrEx – contents of Cu, Fe, Zn, Mn, and Cr in extracts, respectively; CuTr, FeTr, ZnTr, MnTr, and CrTr – transfers of Cu Fe, Zn, Mn, and Cr from green teas to extracts, respectively.
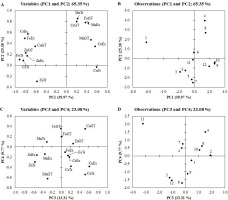
Figure 1B presents distribution of the samples between PC1 and PC2. Tea No. 1 was clearly separated from the rest due to its high Cu and Zn contents, Fe, Cr, Zn content in its extract, as well as high transfer of Cr and Fe. Tea No. 1 was in the form of bags; hence, its manufacturing technology was also an important factor in terms of the final quality of the product. All of the mentioned variables were negatively correlated with PC1 and inter-correlated with each other. The rest of the samples were distributed on the right from tea No. 1 on PCA plot. Position of teas No. 4 and 8 was influenced by their high content of Fe, Mn, and Cr and Mn transfer to the extract.
Figure 1C visualises distribution of variables correlated with PC3 and PC4 which explained 23.08% of the total variance (PC3 – 13.31% and PC4 – 9.77%). These values are not very high, nevertheless contribute to the overall description of the system. Distribution of samples based on PC3 and PC4 is shown in Figure 1D. Position of Tea No. 11, which is separated from other samples, can be justified by low transfer of Cu and high transfer of Zn and Mn to the extract of this tea.
Chromatographic separations of the extracts delivered information about the content of their main compounds, i.e. caffeine, EGC, EC, EGCG, and ECG. The content of the caffeine was in the range of 53.2–70.3 mg/g extract. The flavan-3-ols were quantified in extracts at 56.4–126 mg/g (EGC),26.1–44.4 mg/g (EC), 113–178 mg/g (EGCG), and 38.7–91.9 mg/g (ECG). Their sum was 259–365.4 mg/g extract.In the case of the contents in green teas, values for caffeine,EGC, EC, EGCG, ECG, and total flavan-3-ols ranged wereat 16.1–25.0, 16.6–40.9, 7.28–16.7, 35.0–60.5, 10.8–34.6, and 88.3–148 mg/g, respectively. These results are in accordance with literature data concerning flavan-3-ols of commercially available green teas [Svoboda et al., 2015] and green teas obtained from fresh leaves by various processing methods (roasting, baking, steaming, and sun-drying) [Shi et al., 2022] as well as concerning caffeine of green teas from leaves of wild and cultivated plants [Chowaniak et al., 2021]. In turn, Jiang et al. [2019] reported slightly lower contents of both caffeine (15.6 mg/g) and flavan-3-ols (11.3, 4.5, 27.9, 10.3, and 52.24 mg/g for EGC, EC, EGCG, ECG, and total flavan-3-ols, respectively) in green tea prepared from fresh leaves compared to the values determined in our study.
Flavonoids with multiple hydroxyl groups and with the carbonyl group on ring C in their structures may form complexes with transition metal ions [Kejík et al., 2021; Samsonowicz & Regulska, 2017]. Green tea flavan-3-ols contain catechol and/or galloyl moieties that allow them to bind with iron, zinc, cooper, manganese, chromium, and other metal ions [Bronco et al., 2005; Cherrak et al., 2016; Lee & Heffern, 2022; Navarro et al., 2005]. We postulate that such complexes may affect the transfer of elements from green tea to extracts.
To verify this hypothesis, the contents of individual compounds and total flavan-3-ols determined in green teas and extracts were correlated with contents of elements in green teas and extracts, and with their transfer from the teas to the extracts. The correlation coefficients are shown in Table 3. The most powerful positive correlations were found between Zn transfer to extracts and contents of EGC (r=0.829) and EGCG (r=0.793) in green teas. Consequently, a strong correlation occurred with total flavan-3-ols (r=0.703). High r value was also obtained for a correlation between the content of Zn in extracts and EGC in green teas. In the case of phenolic contents in extracts and Zn variables, there were again significant (p<0.05) positive correlations of EGC with Zn transfer, EGCG with Zn transfer, EGC with Zn content in extract, and additionally, total flavan-3-ols with Zn content in extract. Transfer of iron to extracts was positively (p<0.05) associated with contents of EGC in both extracts and green teas. The correlation between EGC (in both extracts and teas) and Fe content in the extract was also significant (p<0.05). Tolrà et al. [2020] reported that Fe content in tea leaves was positively related to galloylated flavan-3-ols (ECG and EGCG). Contrary to this finding, in our study, correlations between contents of Fe and mentioned phenolics in green teas were not significant (p≥0.05), and moreover, significant (p<0.05) but negative correlations were found between Fe content and EC as well as between Fe content and total flavan-3-ols (Table 3). For Cu and Mn variables, generally, negative correlations were determined with caffeine and phenolic compound contents. Among them, the most powerful were relationships between EC in teas and Mn content in extracts (r=–0.864) and Mn transfer to extracts (r=–0.909). Significant (p<0.05) correlations of the same Mn variables were found with ECG, total flavan 3-ols and caffeine in teas and EC and ECG in extracts. Moreover, Mn content in green teas significantly (p<0.05) negatively correlated with EC (r=–0.598) and EGC (r=–0.579) in teas. A previous study reported a negative (although statistically insignificant) correlation between total phenolic content (TPC) and Mn content in infusions of commercial available green, black, and red teas [Klepacka, 2022]. The author also noted a similar trend for the correlation between TPC and Cu content. In our research, Cu transfer to the extract significantly (p<0.05) negatively correlated with total flavan-3-ols in extracts and positively with caffeine in extracts (Table 3). Moreover, significant (p<0.05) correlations were determined between EGCG in teas and Cu content in the extract (r=–0.795) and its transfer to the extract (r=–0.596). In the case of Cr variables, all correlations were insignificant (p≥0.05).
Table 3
Correlation of contents of Fe, Zn, Cu, Mn, and Cr in green teas (GT) and extracts (EX), and transfer of green tea elements to extracts (TR) with contents of individual flavan-3-ols and caffeine.
Green teas | Green tea extracts | |||||||||||
---|---|---|---|---|---|---|---|---|---|---|---|---|
Caffeine | EGC | EC | EGCG | ECG | Total flavan-3-ols | Caffeine | EGC | EC | EGCG | ECG | Total flavan-3-ols | |
FeGT | -0.500 | -0.453 | -0.586* | -0.565 | -0.468 | -0.690* | -0.042 | -0.221 | -0.486 | -0.219 | -0.396 | -0.566 |
FeEX | -0.359 | 0.460 | -0.119 | 0.003 | -0.084 | 0.063 | -0.576* | 0.587* | -0.110 | 0.122 | -0.050 | 0.414 |
FeTR | -0.099 | 0.592* | 0.161 | 0.207 | 0.136 | 0.339 | -0.500 | 0.600* | 0.125 | 0.137 | 0.130 | 0.567 |
ZnGT | 0.048 | 0.199 | 0.327 | -0.043 | 0.376 | 0.233 | -0.351 | 0.119 | 0.278 | -0.309 | 0.383 | 0.153 |
ZnEX | 0.075 | 0.764* | -0.021 | 0.550 | -0.144 | 0.437 | -0.248 | 0.738* | -0.202 | 0.520 | -0.224 | 0.635* |
ZnTR | 0.536 | 0.829* | 0.237 | 0.793* | -0.010 | 0.703* | 0.027 | 0.598* | -0.106 | 0.445* | -0.203 | 0.526 |
CuGT | -0.403 | 0.070 | 0.099 | -0.199 | 0.273 | -0.006 | -0.667* | 0.187 | 0.219 | -0.147 | 0.374 | 0.276 |
CuEX | -0.411 | -0.627* | -0.364 | -0.795* | -0.318 | -0.744* | 0.314 | -0.355 | -0.020 | -0.403 | -0.164 | -0.559 |
CuTR | 0.046 | -0.524 | -0.084 | -0.596* | -0.155 | -0.469 | 0.614* | -0.449 | 0.121 | -0.518 | -0.097 | -0.629* |
MnGT | -0.302 | -0.113 | -0.598* | -0.210 | -0.579* | -0.444 | 0.240 | 0.127 | -0.520 | 0.239 | -0.506 | -0.137 |
MnEX | -0.696* | -0.209 | -0.864* | -0.303 | -0.747* | -0.666* | -0.099 | 0.158 | -0.725* | 0.370 | -0.633* | -0.144 |
MnTR | -0.801* | -0.113 | -0.909* | -0.275 | -0.830* | -0.672* | -0.242 | 0.275 | -0.752* | 0.421 | -0.730* | -0.093 |
CrGT | -0.509 | -0.172 | -0.550 | -0.435 | -0.448 | -0.531 | -0.001 | 0.134 | -0.370 | 0.005 | -0.342 | -0.154 |
CrEX | -0.366 | 0.322 | -0.142 | -0.129 | -0.073 | -0.037 | -0.460 | 0.478 | -0.075 | 0.012 | 0.002 | 0.312 |
CrTR | 0.028 | 0.395 | 0.288 | 0.109 | 0.266 | 0.314 | -0.358 | 0.335 | 0.261 | -0.077 | 0.277 | 0.371 |
In general, positive significant correlations between the content of flavan-3-ols in the extracts and the content and transfer of Zn and Fe to extracts indicate that the complexes between these metal ions and flavan-3-ols could have been formed and have a significant effect on element transfer to extracts. Although flavan-3-ols are the predominant phenolic compounds [Wong et al., 2022], their polymeric forms (proanthocyanidins) have also been determined in small amounts in green tea [Janiak & Amarowicz, 2018; Shi et al., 2022]. These compounds, due to the multiple catechol and galloyl moieties in the structure, have been shown to exhibit stronger ability to bind metal ions than other classes of phenolics [Karamać & Pegg, 2009]. Moreover, these compounds are extracted with 80% acetone [Janiak & Amarowicz, 2018; Karamać & Pegg, 2009]. Proanthocyanidins could therefore contribute to the transfer of metal ions to the extracts and at the same time disrupt correlations of Fe and Zn contents with flavan-3-ols analysed in our study. This would explain that not all correlations were significant. In turn, positive correlations obtained for Zn and Fe variables and negative one for Cu and Mn variables could suggest their competitiveness to the hydroxyl groups of phenolic compounds. On the other hand, negative correlations shown for Cu and Mn may indicate the lack of interaction of these metal ions with flavan-3-ols and their presence in green teas in species that were not transferred to the extract. Indeed, Pongrac et al. [2020] found that part of manganese was allocated in tea leaf oxalate crystals, which are hardly soluble in water and in organic solvents. Nevertheless, these assumptions still need to be elucidated in future researches.
CONCLUSIONS
The results of this study demonstrate that among the analysed elements, manganese was the most abundant in commercial available green teas, followed by iron, zinc, copper, and chromium. The contents of these elements in green tea extracts were lower, especially for manganese; however, the extracts can still be considered as a significant source of iron, copper, chromium, and zinc. Iron turned out to be the predominant element in extracts. The transfer of elements to the extracts varied considerably. Manganese transfer was by far the lowest. Iron transfer may also be considered low. Comparable values were found for copper, zinc, and chromium. The use of 80% (v/v) acetone allowed obtaining an extract with a high content of flavan-3-ols (EGC, EC, EGCG and ECG) and caffeine. As contents of some of phenolics correlated positively with the Zn and Fe contents in extracts and the transfer of these elements to extracts, it was concluded that flavan-3-ols may positively affect the transfer of iron and zinc from green teas to extracts. Such relationships were not found for the remaining elements. More research is, however, needed to explain the reasons of differences in the transfer of elements from green teas to extracts and of low transfer of some of them. Nevertheless, the green tea extract was proved to represent a rich source of not only bioactive compounds but also trace elements.