INTRODUCTION
Lycopene is the principal carotenoid present in high amount in tomatoes and tomato-derived products. It is an acyclic, open chain, unsaturated carotenoid with 13 double bonds, 11 of which are conjugated and organised linearly [Egydio et al., 2010; Fernandez-Ruiz et al., 2010]. In biological systems, lycopene is the most effective singlet oxygen quencher among carotenoids [Young & Lowe, 2001]. It can remove singlet oxygen atoms two and ten times more efficiently than β-carotene and α-tocopherol, respectively [Przybylska, 2020]. Due to its strong antioxidant potential, lycopene has been shown to provide protection against various types of cancer, including prostate, stomach, and lung [Cheng et al., 2020; Stahl & Sies, 1996], and other forms of oxidative damage. It has also been shown to decrease the risk of chronic diseases including coronary heart disease as well as neurodegenerative diseases such as Alzheimer’s and Parkinson’s diseases [Przybylska, 2020; Willcox et al., 2003]. In addition to its antioxidant capabilities, lycopene induces cell-to-cell contact and modulates the hormonal and immunological systems as well as other metabolic pathways [Cheng et al., 2020; Fuhramn et al., 1997]. Moreover, it is a natural red pigment utilised as a colouring ingredient in the dyeing of various foods [Castro et al., 2021]. Its significance is growing as the food industry uses more natural pigments than synthetic colourants and as law regarding the use of food additives becomes more stringent. Therefore, lycopene is in high demand by pharmaceutical companies and the food, feed, and cosmetic industries.
Tomatoes and tomato products are the primary sources of lycopene in the human diet [Dasgupta & Klein, 2014]. Typically, 80–90% of the total carotenoids in tomato fruit are lycopene [Shi & Maguer, 2000]. Lycopene and other carotenoids are mostly found in the water-insoluble fraction and have shown to be five times more concentrated in the peel and seeds, as compared to the tomato pulp [Toor & Savage, 2005]. Therefore, a large amount of lycopene is lost as waste during tomato processing [Baysal et al., 2000].
The tomato processing industry generates substantial volumes of solid waste, and between 10–40% of the tomatoes processed in a facility are skins and seeds [Al-Wandawi et al., 1985; Topal et al., 2006]. In Vietnam, the production area of tomatoes has been progressively expanding in recent decades for both fresh consumption and processing. Thus, large amounts of low-grade fruit and processing waste are land filled or used as compost and animal feed without significant treatment. Therefore, valorisation of the tomato waste stream by extracting a highly added-value component, such as lycopene, from the waste will contribute to food sustainability and environmental protection. So far, the most common methods for lycopene extraction from plant tissues are based on extraction with organic solvents or supercritical fluid extraction [Deng et al., 2021; Guerra et al., 2021]. The latter requires high capital costs and limits its application to research and industrial scale [Montesano et al., 2008]. The organic solvent extraction method has been more widely utilised due to the lower cost and better lycopene recovery [Pandya et al., 2015].
Vegetable oils are rich in polyunsaturated fatty acids, yet they are extremely vulnerable to oxidation. This results in rancid odours, disagreeable flavours, and discolouration, causing negative alterations in sensory and nutritional qualities and a loss in shelf life [Machado et al., 2022]. Commonly, synthetic antioxidants, such as butylated hydroxyanisole (BHA), butylated hydroxytoluene (BHT), and tert-butyl hydroquinone (TBHQ), are added to edible oils to increase their shelf life. Recent reports indicate these synthetic antioxidants may cause adverse side effects to human health [Mika et al., 2023; Zhang et al., 2023]. Consequently, there is significant interest in enriching oils with natural antioxidants, such as lycopene, tocopherols, polyphenols, to hinder lipid oxidation [Bodoira et al., 2017; Omer et al., 2014; Xie et al., 2018; Zahran & Najafi, 2020].
The goal of this work was to obtain extract rich in lycopene from tomato pomace obtained after pressing the juice and using it to enhance the oxidative stability of peanut oil. Our objectives were to (1) optimise the lycopene extraction process from tomato processing waste using design of experiment (DOE) in combination with a multiple response criterion, and (2) utilise the extract rich in lycopene to enhance the oxidative stability of peanut oil. In this study, peanut oil was chosen to assess the extract’s effect as it is prone to be oxidised due to a relatively high amount of polyunsaturated fatty acids and widely used in the food industry.
MATERIALS AND METHODS
Materials and reagents
The fruits of tomato cv. Savior were manually harvested at the red ripe stage from a commercial field in Nam Dinh province, Vietnam. After harvest, tomatoes were transported under ambient conditions to the food processing laboratory at the Vietnam National University of Agriculture in Hanoi. The fruits were washed, drained, and mechanically pressed to extract the juice. The tomato pomace was dried in a natural convection oven (UN55, Memmert, Schwabach, Germany) at 40°C until the moisture content reached 6.2 g/100 g. The dried pomace was ground using a grindomixer (GM200, Retsch, Haan, Germany). The powder was passed through a 0.15 mm sieve and used for lycopene extraction.
Peanuts were collected from a commercial plantation in Bac Ninh province, Vietnam. Peanut oil extraction was carried out in a single step with a pilot scale screw press. The moisture content of the peanuts averaged 12 g/100 g, the pressing temperature was 28°C, the screw speed was 20 rpm, and the restriction die was 6 mm [Martínez et al., 2012]. The extracted oil was filtered through filter press and stored until use in amber glass bottles in a freezer at −20°C.
The analytical grade reagents included a lycopene standard, 6-hydroxy-2,5,7,8-tetramethylchroman-2-carboxylic acid (Trolox), 2,2-diphenyl-1-picrylhydrazyl (DPPH) radical, butylated hydroxytoluene (BHT), and p-anisidine, all purchased from Sigma-Aldrich (Saint Louis, MO, USA). All other reagents were also of analytical grade and obtained from Merck (Darmstadt, Germany) and Himedia (Mumbai, India). Distilled water of high-performance liquid chromatography (HPLC) grade was used throughout the experiments.
Response surface experimental design for optimisation of lycopene extraction from dried tomato pomace
To identify the potential factors and their working range for optimising lycopene extraction in the current study, the screening experiments were first conducted in which the dried tomato pomace was extracted using various organic solvents at different temperatures and solvent to dried tomato pomace ratios. As the extract will then be added to the edible oil for human consumption, only the solvents with food-grade were tested, i.e., petroleum ether, ethyl ether, ethyl acetate, acetone, and ethanol. From the screening experimental results, ethyl acetate was determined as the most efficient solvent for the next experiments as it gave the highest lycopene extraction yield and economic efficiency and ensured food safety. Therefore, in this study, three independent variables having great influence on the lycopene content and antioxidant activity were retained for optimisation, namely: the ratio of ethyl acetate to material (X1), extraction temperature (X2), and extraction time (X3) using the response surface methodology (RSM). For these three variables, a Box-Behnken design based on 15 trials involving three center points was constructed using the JMP software, version 10.0 (SAS Institute, Inc., Cary, NC, USA) (Table 1). The extraction experiments were carried out randomly to minimise the effect of unexpected variability in the response variables. During extraction, the mixture was stirred using a magnetic stirrer under darkness. After extraction, the extract was analysed for its lycopene content and antioxidant activity. Next, the experimental results of the RSM were fitted with a second-order polynomial equation (Equation (1)) consisting of linear, quadratic and first-order interaction terms by multiple regression.
where: Y is one of the output variables; βi, βii, βij are regression coefficients for the major factors and interaction terms; Xi, Xj are the independent variables; k is the number of variables; and ε is the remaining unexplained error. The multiple-least square regression was used to estimate regression coefficients by minimising the sum of squares of the errors. Using analysis of variance (ANOVA), the significance of the overall model and each regression coefficient was determined. Lastly, response surface optimisation based on the Derringer’s desirability function was used as previously described by Tran et al. [2016] to discover the ideal combination of parameters that maximise the lycopene content and antioxidant activity. The JMP software, version 10.0, was utilised for data analysis (SAS Institute).Table 1
Box-Behnken design matrix of variables (X1, X2 and X3), and measured responses (Y1 and Y2) for lycopene extraction from dried tomato pomace.
Oxidative stability assessment of extract-enriched peanut oil
The oxidative stability assessment of the extract-enriched oil was performed as follows. Lycopene was extracted from dried tomato pomace under the optimised conditions. After removing the solvent using a vacuum evaporator (R-300, Buchi, Flawil, Switzerland) at 40°C and 240 mbar, the extract powder was added to the peanut oil in a range of 1–2 g/kg oil. BHT with concentration of 50 mg/kg oil was used to compare the effect of extract with a synthetic antioxidant. The antioxidants (extract or BHT) were uniformly dissolved in the peanut oil by using a shaker until a homogeneous oil was obtained. The peanut oil without any antioxidant was used as the control. The mixed oils were transferred separately to amber glass bottles containing 1 L of peanut oil and stored in a dark chamber at 40±1°C for up to 60 days. All the enriched and control oil samples were prepared in triplicate and the quality attributes were monitored every 14th day by analysing the peroxide value (PV), p-anisidine value (p-AV), and total oxidation (TOTOX).
Analytical methods
Determination of lycopene content
The lycopene content was quantified by using an LC-10AI HPLC system equipped with an SPD-M20A detector (Shimadzu, Kyoto, Japan). The chromatographic analysis was performed using the method developed by Kimura et al. [2007] with some modifications. Briefly, after tomato dried pomace extraction, a 20 μL aliquot of the extract was injected into an ODS C18 column (4.6×250 mm, 5 μm particle size; Agilent, Santa Clara, CA, USA). The mobile phase comprised distilled water (A), and acetone (B). The combined solvent flow rate was 1.2 mL/min; the column temperature was set at 30°C. The gradient program lasted for 26 min and was as follows: 80% B for 0–7 min; 95% B for 7–20 min; 100% B for 20–25 min; 80% B for 25–26 min. Absorbance was monitored at 470 nm. The lycopene standard was used to establish the calibration curve for quantifying lycopene in the sample. The lycopene content was expressed as mg per g of the pomace dry weight (DW) and per g of the dried extract.
Determination of antioxidant activity
Antioxidant activity of the extract was evaluated based on the scavenging activity of the DPPH radical. The sample preparation was performed according to Martinez & Maestri [2008]. Briefly, reaction mixtures containing 0.1 mL of the extract and 3.9 mL of a DPPH solution at a concentration of 0.1 mM in toluene were incubated for 30 min at room temperature. The absorbance at 515 nm was measured using a UV-VIS DR3900 spectrophotometer (Hach, Ames, IA, USA). The antioxidant activity was calculated using the Trolox calibration curve and expressed as μmol Trolox equivalent (TE) per g pomace DW and per g of the dried extract.
Oil quality analysis
Standard American Oil Chemists’ Society (AOCS) methods were used to determine PV (method Cd 8b-90) [AOCS, 2009] and p-AV (method Cd 18-90) [AOCS, 2017]. TOTOX was derived using the formula (2) [Shahidi & Wanasundara, 2002].
RESULTS AND DISCUSSION
Optimization of lycopene extraction by response surface methodology
The amounts of lycopene extracted from dried tomato pomace at the ratio of solvent to tomato pomace powder of 10:1, 30:1, and 50:1 (v/w) and temperatures of 30, 50, and 70°C for incubation periods up to 150 min are presented in Table 1. The lowest amount of lycopene (4.24 mg/g DW) was extracted at the ratio of 10:1 and a temperature of 30°C. Lycopene in the extract increased sharply from 4.24 to 8.76 mg/g DW when the ratio of solvent to material increased from 10:1 to 30:1 and extraction temperature ranged from 30 to 50°C. The positive effect of temperature on lycopene extraction found in this research is consistent with a previous study by Kumcuoglu et al. [2014] who found that increasing the temperature from 20 to 60°C during solvent extraction of lycopene from tomato processing waste resulted in a 20 mg/kg increase in lycopene content. In addition to temperature, the extraction time significantly contributed to the lycopene content for the duration from 30–90 min, after which no further improvements were detected (Table 1). When the ratio of solvent to solid is high, the solvent more easily diffuses through the tomato tissue and can extract lycopene. Moreover, it creates stronger diffusion dynamics to accelerate the mass transfer and extraction rate, facilitating the release of lycopene. However, further increasing the ratio of solvent to solid to 50:1 did not significantly change the lycopene content, likely because the mass transfer process between solvent solution and solid had reached its maximum. Although increasing temperature accelerates thermodynamic reactions, if the temperature is too high (>70°C) lycopene can lose stability and be degraded due to its long chain of conjugated carbon-carbon double bonds in the molecular structure [Calvo et al., 2007; Ganje et al., 2018].
The antioxidant activity of the extract was also influenced by the ratio of solvent to tomato pomace, temperature, and time in a similar manner as the lycopene content. The highest antioxidant activity of the extract (>12 μmol TE/g DW) was obtained from treatments 8, 13, 14, 15 when the ratio of solvent to waste material was 30:1–50:1, the temperature was 50°C, and the extraction time was 90–150 min (Table 1). The lowest antioxidant activity of 4.54 μmol TE/g DW was obtained when the ratio of solvent to tomato pomace was 10:1 and the temperature was 30°C. The high correlation of lycopene content and antioxidant activity was because lycopene has high antioxidant activity. Generally, a higher lycopene content will provide more antioxidant activity.
The lycopene content obtained in this study is in the same range as that published by Eh & Teoh [2012] in which the yield of all-trans lycopene was 5.11 mg/g DW extracted from tomato using ultrasonic-assisted extraction. Lavecchia & Zuorro [2008] obtained a lower lycopene content (450 mg/100 g DW) using cell wall degrading enzymes during the extraction process. Silva et al. [2019] extracted the lycopene from tomato processing by-product with innovative hydrophobic eutectic mixture (dl-menthol as a hydrogen-bond acceptor (HBA) and lactic acid as a hydrogen-bond donor (HBD)) using ultrasound-assisted extraction. Under the optimal extraction condition of 8:1 mol HBA to mol HBD ratio, solvent to sample ratio of 120 mL/g, at 70°C for 10 min, the optimal yield of lycopene was 1446.6 μg/g DW. Kehili et al. [2019] showed the highest lycopene content of 1,223.72 mg/kg DW obtained when refined olive oil was used as the extractant, and a biomass to oil ratio was 2.5% (w/v), temperature was 80°C, and magnetic stirring was continued at 400 rpm for 45 min. The higher lycopene content obtained in the present study might be due to differences in tomato source, moisture content, and solvent extraction method. Tomato lycopene content varies depending on cultivar, maturity stage, and agronomic and environmental conditions during growth [Sumalan et al., 2020; Taoukis & Assimakopoulos, 2010].
To evaluate the effect of three experimental factors on lycopene content (Y1) and antioxidant activity (Y2), the experimental results were fitted to the quadratic equation given in Equation (1). Table 2 presents the fitting results for each response variable. The models were significant for both lycopene and antioxidant activity (p<0.0001). The coefficient of determination (R2) was 0.9872 for lycopene content and 0.9703 for antioxidant activity, demonstrating that 98.72% and 97.03% of the variability in these response variables could be explained by the model. Interestingly, only the second-order interactions X1×X3 for lycopene content and X1×X3 and X2×X3 for antioxidant activity were statistically significant at the 5% level, all having a positive effect (Table 2). On the contrary, all the quadratic terms (X12, X22, X32) were significant and negatively related to the response variables. In other words, excessive high temperature and longer extraction time may lead to lycopene degradation and isomerisation [Ganje et al., 2018]. The results found in this study are consistent with those published by Dhakane-Lad & Kar [2021] who reported a serious loss of lycopene from pink grapefruit when supercritical extraction was conducted at temperature above 74°C.
Table 2
Coefficients of the second-order models for lycopene content and antioxidant activity of the lycopene extraction from dried tomato pomace.
Components | Lycopene content (mg/g DW) | Antioxidant activity (μmol TE/g DW) |
---|---|---|
Intercept | 3.482 | 3.416 |
Ratio of solvent:material (X1) | 0.035 | 0.071 |
Temperature (°C) (X2) | 0.074 | 0.112 |
Time (min) (X3) | 0.005 | 0.013 |
X12 | −0.002 | −0.004 |
X1×X2 | 0.0001* | 0.0004* |
X22 | −0.004 | −0.009 |
X1×X3 | 0.0002 | 0.0003 |
X2×X3 | 6.04×10−5* | 0.0006 |
X32 | −0.0015 | −0.0003 |
Model (p-value) | −0.0015 | <0.0001 |
R2 | 0.9872 | 0.9703 |
Figure 1 illustrates the graphical depiction of the global desirability function for optimising the lycopene content, while Figure 2 depicts the three-dimensional representations of the global function in the space of the distinct variables. However, these are only partial representations, as one of the three main factors was held constant at its optimal value. Their optimal areas were comparable for various extraction factor combinations, reaching 9.09 mg/g DW for lycopene content and 12.99 mol TE/g DW for antioxidant activity.
Figure 1
Three-dimensional response surface plots showing the effect of solvent to dried tomato pomace ratio vs. temperature (A), solvent to tomato dried pomace ratio vs. time (B), and temperature vs. time (C) on lycopene content.
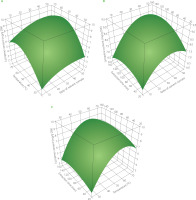
Figure 2
Three-dimensional response surface plots showing the effect of solvent to dried tomato pomace ratio vs. temperature (A), solvent to dried tomato pomace ratio vs. time (B), and temperature vs. time (C) on antioxidant activity.
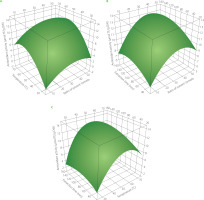
To determine the optimal values for the three main factors of the extraction process that maximise the lycopene yield and antioxidant activity, these output variables served as new variables for the desirability function to gain the global desirability score. The optimisation results indicated that the maximum lycopene content and antioxidant activity were achieved when lycopene extraction process was carried out at the ratio of ethyl acetate to tomato material of 35:1 (v/w), at 55°C for 100 min (Figure 3). After that, the effectiveness of the predicted regression was assessed by three replicate experiments conducted under the optimised conditions. The extraction efficiency of the samples was in line well (98.6%) with that predicted by the RSM experiments. The extract was then used to evaluate the oxidative stability of peanut oil.
Oxidative stability assessment of lycopene-enriched peanut oil during storage
Lycopene-rich powder was obtained by extraction under the optimal conditions, i.e., ratio of ethyl acetate to tomato pomace of 35:1 (v/w), at 55°C for 100 min, followed by solvent evaporation prior to its addition to the peanut oil. The dried extract had the lycopene content and antioxidant activity of 0.89 g/g, and 0.82 mmol TE/g respectively. The oxidative stability of the untreated and antioxidant-enriched oils is presented in Table 3. During storage, the oxidative indexes, including PV, p-AV, and TOTOX, increased in all treatments. The oil oxidation process is proportional to the storage time. However, within one storage time point, there were variations in oxidation for the different treatments. Specifically, the untreated oil (control sample) always had the highest PVs while the peanut oil with 2 g extract/kg oil had the lowest levels during 60 days of storage. There were no significant differences (p≥0.05) in PVs between the oils with 50 mg BHT/kg oil and those with 1 g extract/kg oil on days 14, 32, 46, and 60, except for day 28 when the extract added peanut oil had a significantly lower PV than BHT added peanut oil. Low PVs in the extract-enriched oil indicate the effective antioxidant activity of lycopene in retarding primary oxidation to form peroxide compounds. According to the Codex Alimentarius standard 210-1999 [CODEX STAN, 2015], PV for cold-pressed and virgin oils is allowed up to 15 meq O2/kg oil. Therefore, after 60 days of storage, peanut oils with BHT or lycopene-rich extract of 1 g/kg oil and 2 g/kg oil were still acceptable, as their PVs were 9.76, 9.83, and 8.55 meq O2/kg respectively. The untreated oil with PV of 16.29 meq O2/kg exceeded the threshold value. Data obtained in the current research are consistent with those published by Omer et al. [2014] in which lycopene-rich extract at the concentration of 1,000 ppm was proved to retard lipid oxidation in the refined sunflower oil. Li et al. [2011] also demonstrated the positive effect of lycopene at the concentration of 0.01% on oxidative stability of soybean oil as determined by measuring the peroxide value and acid value.
Table 3
Quality indexes of peanut oil without (control) and with the extract from dried tomato pomace during storage at 40°C.
[i] Data values are accompanied by standard deviation of the mean with n=3. Within a column, separately for each index, results with the same letter were not significantly different (p≥0.05). TOTOX, total oxidation; BHT, peanut oil with butylated hydroxytoluene addition of 50 mg/kg oil; L1, peanut oil with extract addition of 1 g/kg oil; L2, peanut oil with extract addition of 2 g/kg oil.
The p-AV generally shows the magnitude of aldehydic secondary oxidation products thus, it is an indicator of the freshness of vegetable oil. Results showed that at every storage time, the p-AVs of the control sample were significantly (p<0.05) higher compared to those of the oils with synthetic antioxidant (BHT) or extract addition (Table 3). The p-AVs of the BHT-added oil were statistically different (p<0.05) from those with 1 g extract/kg oil at the end of storage time. The oil with 2 g extract/kg oil had the lowest oxidation level and its p-AVs reached 2.02 after 60 days of storage. The p-AV of peanut oil with the addition of lycopene-rich extract analysed in the current study was higher than that of linseed oil with 80 mg lycopene extract/kg oil whose value only reached 0.23 [Condori et al., 2020]. Zahran & Najafi [2020] reported the positive effect of olive leaves extract at concentrations of 1,000 and 1,500 ppm on reducing the p-AV in refined soybean oil; however, its value was much higher than that in our study, reaching 23.0 after 21 days of storage at 60°C. The variation in p-AVs could be due to differences in antioxidant activity of the extracts, oil properties, and storage conditions.
TOTOX, an indicator of the oxidative state of the oil, was calculated from the PV and p-AV, therefore its changes follow a similar trend to PV and p-AV. The untreated oil had the highest oxidation level with the TOTOX value increasing from 8.68 on day 0 to 36.24 on day 60. While the peanut oil stabilized with 2 g extract/kg oil had the smallest value of 19.12 on day 60. This was a similar level to the control sample on day 28, indicating it was the most stable, followed by the oils with 50 mg BHT/kg and with 1 g extract/kg oil. Our findings were consistent with the study by Chong et al. [2015], which indicated that 200 ppm mangosteen peel extracts exhibited superior reduction in the TOTOX value in sunflower oil compared to 200 ppm BHT after 24 d of storage at 65°C.
CONCLUSIONS
The results clearly demonstrated the effect of the ratio of ethyl acetate to tomato waste, extraction temperature, and time of extraction on the lycopene content and antioxidant activity of the extract. By using response surface methodology, it was possible to identify the optimal extraction condition for lycopene from tomato dried pomace. This was estimated to be the ratio of solvent to tomato waste material of 35:1 (v/w), at 55°C for 100 min. Addition of 2 g extract/kg oil to the peanut oil significantly enhanced its oxidative stability. Incorporation of lycopene from tomato by-products may extend the shelf-life and improve the acceptability and valorisation of edible oils.