INTRODUCTION
Soy protein isolate (SPI), together with soy protein concentrate (SPC) and soy flour, is a renowned commercial product of processed soybean (Glycine max) [Qin et al., 2022]. Containing a minimum of 90 g of protein in 100 g with two major water-insoluble globulins (glycinin or 11S and β-conglycinin or 7S), SPI is commonly used in nutritional drinks, protein bars, and infant formula products [Paulsen, 2009; Qin et al., 2022]. Health benefits of soy protein consumption, including prevention of cancer, reduction of cardiovascular disease-associated risks and complications, lowering low-density lipoprotein cholesterol, and improvement of postmenopausal symptoms in women have been reported in a number of studies [Lima et al., 2017; Messina, 2014; Qin et al., 2022; Sirtori et al., 2009]. However, the digestibility of soy protein is lower than that of other protein sources; its in vitro digestibility value is often less than 80%. This is due to the presence of antinutritional factors, such as lipooxygenase, urease, and trypsin inhibitors [Vagadia et al., 2017]. In order to increase the protein digestibility, enzymatic hydrolysis has been widely implemented as small peptides in the protein hydrolysates can be rapidly absorbed by small intestine [Webb Jr, 1990]. Enzymatic hydrolysis is also more desirable than chemical hydrolysis due to its advantages in reducing side chemical reactions and unwanted products [Campbell et al., 1996]. SPI hydrolysates were proven to have higher bioactivities compared to SPI, including anti-adipogenic, anti-angiotensin, anti-inflammatory, anticancer, antioxidant, and antibacterial effects [Hsieh et al., 2022; Joo et al., 2004; Lee et al., 2008; Tsou et al., 2010; Zhang et al., 2020b]. In addition, enzymatic protein treatment also fosters great potential in decreasing soy protein-related allergenicity while improving emulsifying capacity, foaming property, solubility, water- and oil-binding capacity, and sensory profile of SPI hydrolysates relative to the parent proteins [Meinlschmidt et al., 2016].
Commercial enzymes used in SPI hydrolysis are isolated from fungal, bacterial, animal, and plant sources. Since the peptide diversity and consequent bioactivities of hydrolysed products can be restricted with the application of a single type of enzyme, enzyme combination has been considered as a means to obtain SPI hydrolysates with appealing properties, such as antioxidant capacity [Chen et al., 2020]. The use of more than two enzymes or a mixture of native proteases from fruit juices in SPI hydrolysis has not been intensively studied, although it was described for soy proteins and other protein sources [Tovar-Jiménez et al., 2021; Utami et al., 2019]. In one study, soy proteins digested by pineapple juice produced a hydrolysate as a complex nitrogen source for the growth of lactic acid bacteria [Utami et al., 2019]. In another study, a hydrolysate of whey protein concentrate produced by a combination of purified Sporisorium reilianum aspartyl protease (Eap1), chymotrypsin (C), and trypsin (T) (Eap1-C-T) showed antihypertensive activity; however, its capacity was weaker than of that produced by single enzymes (Eap1, C, and T) and combinations of two enzymes (Eap1-C, Eap1-T, and C-T) [Tovar-Jiménez et al., 2021].
Papaya (Carica papaya) and cantaloupe (Cucumis melo) are promising sources of proteolytic enzymes [Kaneda et al., 1997; Pendzhiev, 2002]. The crude papaya extract contains numerous proteases in which a well-known enzyme, papain (EC 3.4.22.2), can be used to generate protein hydrolysates with less pronounced bitterness [Humiski & Aluko, 2007; Meinlschmidt et al., 2016]. Recently, papaya juice has been employed to hydrolyse edible bird’s nest and the achieved hydrolysate has been shown to inhibit α-glucosidase, a hyperglycemia-associated enzyme, whereas the hydrolysates obtained with two commercial enzymes, Alcalase and papain, did not exhibit this ability [Zulkifli et al., 2019]. This suggests the improvement of antihyperglycemic activity in edible bird’s nest hydrolysed by papaya juice as compared to the tested enzymes. Similar to papaya fruit, high amounts of proteases, including a renowned serine protease, cucumisin (EC 3.4.21.25), are present in melon fruit [Yamagata et al., 1989]. A crude melon extract was also reported to produce kilka fish protein hydrolysate with higher antioxidant activity than Alcalase [Alavi et al., 2019].
Due to the proteolytic potentials of papaya and cantaloupe, this study aimed to exploit the ability of two fruit juices in SPI hydrolysis. The bioactivities of the obtained hydrolysates, including in vitro antioxidant, anti-tyrosinase, and wound-healing abilities on fibroblast model, were also compared to those of unhydrolysed SPI and the hydrolysate obtained using the commercial enzyme – papain. The antioxidant capacity was selected for the assessment as this property has been investigated for SPI hydrolysates in various studies [Chen et al., 2020; Kneevic-Jugovic et al., 2023; Lee et al., 2008; Peñta-Ramos & Xiong, 2002]. Moreover, antioxidant peptides derived from the protein hydrolysates have captured great attention for their effects against oxidative stress-related diseases [Zhu et al., 2022]. Recently, researchers have shown interests on the anti-tyrosinase and wound-healing activities of the protein hydrolysates due to their potential application in health and beauty products [Chotphruethipong et al., 2021; Wong et al., 2018]. As a result, the present study evaluated these bioactivities in SPI hydrolysates obtained by fruit juices although they have not been previously recorded for SPI hydrolysates.
MATERIALS AND METHODS
Materials
SPI powder (90 g of protein/100 g of SPI) and papain enzyme (50 USP/mg) were purchased from Shiv Health Foods LLP (Kota, India) and Biogreen BPC., JSC (Hanoi, Vietnam), respectively. Fresh ripe papaya and cantaloupe fruits used in this study were harvested during the wet and dry seasons in Southern Vietnam. Human fibroblast cells were a kind gift from Dr. Tran Le Bao Ha, Laboratory of Tissue Engineering and Biomedical Materials, University of Science, HCMC National University (Ho Chi Minh, Vietnam).
Preparation of papaya and cantaloupe fruit juices and determination of their proteolytic activity
Papaya and cantaloupe were peeled and squeezed; the resultant juices were then centrifuged at 4,951×g for 10 min at 10°C. The supernatants, designated here as papaya juice (PJ) and cantaloupe juice (CJ), were used in the subsequent experiments.
The analysis of the proteolytic activity of PJ and CJ was conducted according to the protocol for the Sigma’s non-specific protease activity assay [Cupp-Enyard, 2008]. Free tyrosinases produced by the proteases of PJ and CJ on the casein substrate were reacted with Folin-Ciocalteau phenol reagent to form blue chromophores, which absorbance was measured by a spectrophotometer (Genway Biotech, San Diego, CA, USA). A standard curve plotted for tyrosine was used to express the protease activity of PJ and CJ in terms of unit, which was defined as the μmol of tyrosine equivalents released from casein per min. The results were calculated by the Equation (1):
where: a is total volume of assay (11 mL), b is time of assay (10 min), c is volume of PJ or CJ (1 mL), and d is volume used in spectrophotometric measurement (2 mL).Preparation of soy protein hydrolysates by papaya and cantaloupe fruit juices, and papain
The SPI slurry (~20 mg/mL) was prepared by mixing 2.0 g of SPI with 100 mL of distilled water. To obtain SPI hydrolysates, a volume of 2.5 mL of PJ or CJ was added to the SPI slurry containing 2.0 g of SPI (juice to substrate ratio of 2.5:2, v/w). The temperature was set at 55°C and the pH was about 6.5 for PJ and 8.5 for CJ during the hydrolysis. The hydrolysed samples after 1, 2, and 4 h of treatment were heated at 90°C for 20 min to terminate the reaction and then centrifuged at 8,801×g for 10 min at 10°C. The obtained supernatants (hydrolysates) were collected for sodium dodecyl-sulfate polyacrylamide gel electrophoresis (SDS-PAGE) analysis and further experiments. The hydrolysates of SPI by PJ and CJ were hereafter designated as HPJ and HCJ, respectively. Mixtures of SPI with papaya and cantaloupe juices were considered as HPJ 0 h and HCJ 0 h, respectively.
The hydrolyses of SPI by papain were carried out at different papain to substrate ratios (0.3125:2, 0.625:2, and 1.25:2, w/w) and the conditions of 55°C, 4 h, and pH 6.5. Subsequent steps were performed similarly to those mentioned above for the SPI hydrolysis with PJ and CJ. The hydrolysate of SPI with papain was hereafter designated as Hpapain. A mixture of SPI and papain was considered as Hpapain 0 h.
Sodium dodecyl-sulfate polyacrylamide gel electrophoresis analysis
Samples including SPI, HPJ 0 h, HCJ 0 h, HPJ and HCJ after 1–4 h of hydrolysis, and Hpapain obtained using different papain to substrate ratios, were mixed with sodium dodecyl-sulfate (SDS) protein loading buffer, boiled for 5 min and loaded on SDS-polyacrylamide gel electrophoresis (SDS-PAGE) separating gels (12.5% of acrylamide, w/v). Color pre-stained protein ladder (New England Biolabs, Ipswich, MA, USA) was used as a molecular weight standard. After running in an electrophoresis apparatus (Bio-Rad Laboratories, Hercules, CA, USA), the gels were stained with Coomassie blue dye to observe protein bands. The SDS-PAGE profile of SPI was analysed according to a previous report [Xia et al., 2020]. The amount of degraded β-conglycinin subunits (α, α’) (in the molecular weight range of 70–100 kDa) was calculated based on the intensity of protein bands using Image J software (version 1.45, National Institutes of Health, Bestheda, MD, USA) in order to relatively compare the hydrolysis of SPI by PJ and papain.
Determination of degree of hydrolysis
The degree of hydrolysis (DH) was determined using o-phthalaldehyde (OPA) reagent according to the previous description [Nielsen et al., 2001]. In brief, aliquots of 25 μL of the samples (SPI, HPJ 0 h, HCJ 0 h, HPJ and HCJ after 1–4 h of hydrolysis, and Hpapain obtained using different papain to substrate ratios) and serine as a standard (0.9516 meqv/L) were pipetted into a 96-well Optiplate (PerkinElmer, Waltham, MA, USA). A volume of 200 μL of freshly prepared OPA reagent was pipetted into each well. The plate was incubated in the dark at room temperature for 5 min and absorbance readings were taken at 340 nm. MilliQ water was used as the blank.
The DH was calculated using Equation (2):
where: H is the number of cleaved peptide bonds during hydrolysis and Htot is the total number of substrate peptide bonds (for soy protein products, Htot = 7.8 meqv/g protein) [Nielsen et al., 2001].The H was calculated based on absorbance of reaction mixture with sample (Asample), serine (Astandard), and blank (Ablank) using Eqations (3) and (4) [Nielsen et al., 2001]:
where: α and β are constant values (0.97 and 0.342 for soy proteins, respectively), C is protein concentration in sample solution (18 g/L), and 0.9516 (meqv/L) corresponds to equivalent weight of the serine standard.Determination of 2,2′-azino-bis(3-ethylbenzothiazoline-6-sulfonic acid) radical cation scavenging capacity
The antiradical capacity of SPI, HPJ, HCJ, and Hpapain (enzyme to substrate ratio of 0.625:2, w/w) from the hydrolysis at 0 and 4 h was performed using 2,2′-azino-bis(3-ethylbenzothiazoline-6-sulfonic acid), ABTS (Sigma-Aldrich Pte. Ltd., Singapore) as previously described [Re et al., 1999]. A volume of 100 μL of diluted SPI (0.625, 1.25, 2.5, 5, 10, and 20 μg/mL) was mixed with 900 μL of ABTS•+ solution and incubated for 15 min. Similar steps were also performed with 100 μL of diluted HPJ, HCJ, and Hpapain 0 h and 4 h containing 0.625, 1.25, 2.5, 5, 10, and 20 μg/mL of initial SPI. The absorbance was measured at 734 nm in a UV spectrophotometer (Genway Biotech, USA). The ABTS•+-scavenging capacity was calculated by the Equation (5):
where: A1 is absorbance of negative control (ABTS•+ solution only), A2 is absorbance of the sample after reacting with ABTS•+, and A3 is absorbance of the blank (the sample without ABTS•+).The concentration of sample which inhibits 50% of the ABTS•+ (IC50) was calculated by GraphPad Prism software (version 5.0, Insightful Science LLC, San Diego, CA, USA).
Determination of hydroxyl radical-scavenging capacity
The assay with hydroxyl radical (•OH) generated in Fenton reaction was carried out following a previous study with slight modifications [Chong et al., 2022]. The initial solution was prepared by mixing 0.5 mL of 9 mM iron (II) sulphate (FeSO4) in 10 mM of ethylenediaminetetraacetic acid, disodium salt (Na2EDTA) and 1.0 mL of 8 mM hydrogen peroxide (H2O2). Then, a volume of 1.0 mL of the diluted SPI, HPJ, HCJ, and Hpapain (enzyme to substrate ratio of 0.625:2, w/w) from the hydrolysis at 0 and 4 h containing 0.125, 0.25, 0.5, 1.0, 2.0, 4.0, 8.0 and 16.0 μg/mL of initial SPI was added into the mixture. Milli-Q water was used as a negative control. Subsequently, 0.2 mL of 9 mM of salicylic acid was added. All test tubes were incubated at 37°C for 30 min and absorbance was read at 510 nm using a microplate spectrophotometer (VICTOR Nivo 3F, PerkinElmer, USA). •OH-scavenging capacity was calculated using the Equation (6):
where: A1 is absorbance of negative control, A2 is absorbance of the sample after reacting with salicylic acid and A3 is absorbance of the blank (the sample without salicylic acid).The IC50 values were calculated by GraphPad Prism software (version 5.0, Insightful Science LLC, USA).
Determination of in vitro wound-healing activity
The in vitro wound-healing assay on the fibroblast model was conducted following the previous report [Nguyen et al., 2023]. In brief, fibroblast cells were cultured in high-glucose Dulbecco’s Modified Eagle Medium (DMEM) (HyClone, Cytiva, Marlborough, MA, USA) supplemented with fetal bovine serum (FBS, 0.1 g/mL) (HyClone, USA) for 24 h at 37°C with CO2 (50 mL/L). After making a wound by a 10 μL pipette tip, the old medium was replaced with high-glucose DMEM medium and test samples (5 mL of sample in 100 mL of medium) including SPI, HPJ, HCJ, and Hpapain (enzyme to substrate ratio of 0.625:2, w/w) from the hydrolysis at 0 and 4 h. Distilled water was used as a negative control. Wound images at 0, 12, and 24 h of treatment were taken in a light microscope (Euromex, Arnhem, Netherlands). The wound areas were quantified using ImageJ software (version 1.45, National Institutes of Health, USA). The wound-healing activity of the samples was calculated by the Equation (7):
where: C1 is the wound area of the sample at 0 h and C2 is the wound area of the sample after 12 and 24 h of treatment.Determination of mushroom tyrosinase inhibitory capacity
The anti-tyrosinase assay using mushroom tyrosinase and l-tyrosine (substrate) (Sigma-Aldrich Pte. Ltd., Singapore) was performed as previously described with small modifications [Tomita et al., 1990]. A volume of 10 μL of tyrosinase (250 U/mL) was added to 60 μL of diluted SPI, HPJ, HCJ, and Hpapain (enzyme to substrate ratio of 0.625:2, w/w) from the hydrolysis at 0 and 4 h containing 1.9, 3.8, 7.5, 15, 30, and 60 μg/mL of initial SPI in a 96-well plate. The plate was incubated at room temperature for 20 min. Then, 140 μL of a reaction medium consisting of l-tyrosine (0.3 mg/mL) in phosphate buffer (pH 6.8) was added to the wells. The formation of l-dopachrome was monitored by measuring the absorbance at 480 nm in a microplate reader (VICTOR Nivo Filter, PerkinElmer, USA). The tyrosinase inhibitory capacity of the samples was calculated using the Equation (8):
where: B1 is absorbance of negative control, B2 is absorbance of the sample after reacting with tyrosinase, B3 and B4 are absorbances of the blank for the negative control and sample, respectively (the reaction without l-tyrosine substrate).GraphPad Prism software (version 5.0, Insightful Science LLC, USA) was used to calculate the IC50 values.
RESULTS AND DISCUSSION
The hydrolysis of soy protein isolate by papaya and cantaloupe juice proteases, and papain
Proteolytic enzymes are present in papaya and cantaloupe [Kaneda et al., 1997; Pendzhiev, 2002]. In order to confirm our obtained juices contained these enzymes, the non-specific protease activity of PJ and CJ was determined by using casein as a substrate. The activity of total proteases in PJ and CJ was 0.87±0.04 U/mL and 2.66±0.02 U/mL, respectively, indicating that proteases were extracted into the juices and might be responsible for the proteolytic activity of the juices.
The SDS-PAGE separations of SPI and hydrolysates are shown in Figure 1. Proteins with molecular weights in the range of 25 kDa to 100 kDa predominated in the SPI profile. Major bands corresponded to subunits of β-conglycinin (α, α’, and β): 70–100 kDa for the α, α’ subunits and approximately 55 kDa for the β subunit. The bands in the range of 25–55 kDa were identified as acidic subunits of glycinin (A1–A4) while the basic subunit had a molecular weight of 25 kDa [Xia et al., 2020]. This SDS-PAGE profile of SPI was consistent with that reported by Xia et al. [2020], although there was a small difference in the distribution of bands resulting in a slight difference in the estimation of protein molecular weight.
Figure 1
SDS-PAGE separation of soy protein isolate (SPI) hydrolysed by papaya juice (PJ), cantaloupe juice (CJ), and papain. (A) and (B) SPI hydrolysed by PJ and CJ at the juice to substrate ratio of 2.5:2 (v/w), temperature of 55°C, and reaction time of 0, 1, 2, and 4 h. (C) SPI hydrolysed by PJ (juice to substrate ratio of 2.5:2, v/w) and papain (enzyme to substrate ratio of 0.3125:2, 0.625:2, and 1.25:2, w/w) at a temperature of 55°C and reaction time of 4 h. (D) Quantification of β-conglycinin subunit (α, α’) degradation (in the red-dashed box in C graph). Each data bar is presented as mean and standard deviation of three independent experiments. Different letters above bars indicate significant differences among samples (p≤0.05).
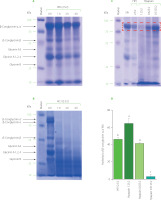
The SDS-PAGE profiles of hydrolysates obtained by PJ showed that after 1 h of SPI treatment with PJ at the juice to substrate ratio of 2.5:2 (v/w), band distribution was comparable to that for unhydrolysed soy proteins (Figure 1A). The proteins began to be significantly degraded after 2 h; however, they were not fully hydrolysed even after 4 h of treatment. This trend in the extent of SPI hydrolysis by PJ was confirmed by determining the degree of hydrolysis. The DH was 5% after 1 h of hydrolysis whereas values reached nearly 10 and 11% after 2 and 4 h of hydrolysis, respectively (Figure 2A).
Figure 2
The degree of hydrolysis of soy protein isolate (SPI) hydrolysed by papaya juice (PJ), cantaloupe juice (CJ), and papain. (A) SPI hydrolysed by PJ and CJ at the juice to substrate ratio of 2.5:2 (v/w), temperature of 55°C, and reaction time of 0, 1, 2, and 4 h. (B) SPI hydrolysed by PJ (juice to substrate ratio of 2.5:2, v/w) and papain (enzyme to substrate ratio of 0.3125:2, 0.625:2, and 1.25:2, w/w) at a temperature of 55°C and reaction time of 4 h. Each data bar is presented as mean and standard deviation of three independent experiments. Different letters above bars indicate significant differences among samples (p≤0.05).
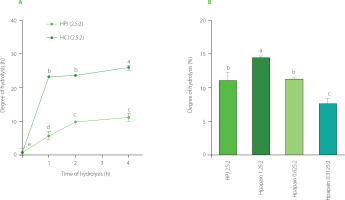
Meanwhile, as seen in the electrophoregrams of SPI hydrolysates obtained by CJ, at the juice to substrate ratio of 2.5:2 (v/w), most of the bands corresponding to high molecular weights disappeared after 1–2 h of hydrolysis indicating that substantial amount of proteins was hydrolysed (Figure 1b). Protein degradation was even more extensive after 4 h of CJ treatment. Consistently, 1 and 2 h of SPI hydrolysis with CJ resulted in a DH of about 23%, while a DH of 26% was obtained after 4 h of hydrolysis (Figure 2b). A crude melon extract produced at the juice to substrate ratio of 2:100 (w/v) was also reported to provoke an extensive hydrolysis on kilka fish protein [Alavi et al., 2019]. This property of melon is attributed to cucumisin (about 10 g in 100 g of crude proteins) and other proteases detected in the fruit juice [Yamagata et al., 1994].
The SDS-PAGE separations (Figure 1) and DH values (Figure 2) showed that the SPI hydrolysis performed by CJ was more extensive than that by PJ at the same juice to substrate ratio of 2.5:2 (v/w). This might be due to the difference in proteolytic activity of these two fruit juices, which was approximately 3-fold higher for CJ than PJ.
In order to compare bioactivities of SPI hydrolysates produced using fruit juices and a commercial enzyme, papain was selected as it is a well-characterised enzyme found naturally in raw papaya fruit [Mamboya & Amri, 2012]. Papain was also employed to produce SPI hydrolysate with better foaming properties and a less bitter taste than Alcalase, Corolase, Neutrase, Protamex, and pepsin [Meinlschmidt et al., 2016]. We hither attempted to find the ratio of papain to substrate which allows for obtaining a relatively similar degree of SPI hydrolysis as when using PJ in the ratio of 2.5:2 (v/w). According to the pattern on the SDS-PAGE gel (Figure 1C) and the DH values (Figure 2b), SPI was the most extensively hydrolysed by papain at the enzyme to substrate ratio of 1.25:2, w/w (DH of nearly 15%). The effective hydrolysis of soy proteins was also observed in a former study using papain at the enzyme to substrate ratio of 1:100 (w/v) [Peñta-Ramos & Xiong, 2002]. The hydrolysis of SPI by papain at the enzyme to substrate ratio of 0.625:2 (w/w) approximately corresponded to that by PJ at juice to substrate ratio of 2.5:2 (v/w), as indicated by the decrease of intensity of the major protein band with the molecular weight in the range of 70–100 kDa (α, α’ subunits of β-conglycinin) and the DH value (approximately 11%) (Figure 1C, 1D, and 2C). The band in the range of 70–100 kDa was selected for quantification since its high molecular weight prevented the overlap with bands of hydrolysis products.
As observed on the SDS-PAGE gel, the profile of hydrolysis products in the PJ-treated sample was dissimilar from that in the papain-treated one (Figure 1C). This is possibly related to the different substrate specificity of native proteases in PJ, resulting in the degradation of proteins at various positions and eventually generating products of hydrolysis with lower molecular weights. The different profiles between two hydrolysates by PJ and papain might contribute to differences in their bioactivities.
Antioxidant capacity of soy protein hydrolysates
Soy protein hydrolysates obtained by protease treatment can possess promising antioxidant property [Chen et al., 2020 ; Kneevic-Jugovic et al., 2023; Lee et al., 2008; Peñta-Ramos & Xiong, 2002]. Therefore, our study investigated if the SPI hydrolysates obtained by PJ, CJ, and papain also exhibited antioxidant capacity.
The results of ABTS assay showed that at the initial SPI concentration of 10 μg/mL, SPI hydrolysates obtained by PJ and CJ after 4 h hydrolysis (HPJ 4 h and HCJ 4 h) were able to scavenge about 95% of ABTS•+ radicals whereas it was only 20% for unhydrolysed SPI and 25% for mixtures of SPI and fruit juices (HPJ 0 h and HCJ 0 h) (Figure 3A). At the same concentration, the ABTS•+-scavenging capacity of hydrolysate obtained by papain after 4 h of hydrolysis (Hpapain 4 h) was approximately 80% higher than that of unhydrolysed SPI (20%) and a mixture of SPI and papain (Hpapain 0 h) (35%). In the •OH-scavenging capacity analysis, HPJ 4 h and HCJ 4 h inhibited about 75% of the •OH, which are more effective than unhydrolysed SPI (nearly 17%), HPJ 0 h, and HCJ 0 h (nearly 40%) at the initial SPI concentration of 16 μg/mL (Figure 3b). At this initial concentration of SPI, the hydrolysate obtained by papain also had higher •OH-scavenging capacity (45%) than Hpapain 0 h (35%).
Figure 3
(A) ABTS•+-scavenging capacity and (B) •OH-scavenging capacity of soy protein isolate (SPI), hydrolysates obtained by papaya juice (HPJ 4 h), cantaloupe juice (HCJ 4 h), and papain (Hpapain 4 h) and unhydrolysed mixture of SPI with papaya juice (HPJ 0 h), cantaloupe juice (HCJ 0 h), and papain (Hpapain 0 h). SPI hydrolysates were prepared at the juice to substrate ratio of 2.5:2 (v/w) or the papain to substrate ratio of 0.625:2 (w/w), temperature of 55°C, and reaction time of 4 h. Each data bar is shown as mean and standard deviation of three independent experiments.
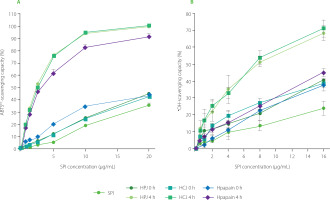
In general, the ABTS•+ and •OH-scavenging capacities of HPJ 4 h (IC50 of 2.39 and 7.17 μg/mL, respectively), HCJ 4 h (IC50 of 2.46 and 6.93 μg/mL, respectively), and Hpapain 4 h (IC50 of 2.75 and 17.85 μg/mL, respectively) were significantly higher in comparison to those of unhydrolysed SPI (IC50 of 24.93 and 42.82 μg/mL, respectively). Our data also revealed a slightly stronger •OH-scavenging capacity of HPJ 4 h than Hpapain 4 h, despite both hydrolysates showed relatively similar DH. This might be rooted from the varying specificity of native proteases in the PJ which released a higher amount of peptides with •OH-scavenging activity or more active peptides. It should be noted that IC50 values of HPJ 0 h, HCJ 0 h, and Hpapain 0 h for the •OH-scavenging activity were slightly lower than that of SPI (Table 1). This higher radical scavenging capacity might be explained by the antioxidant activity of polyphenols of the papaya, carotenoids and vitamin C in the cantaloupe, or papain itself [Fundo et al., 2018; Insanu et al., 2022; Manosroi et al., 2014].
Table 1
IC50 values (μg/mL) of soy protein isolate (SPI), hydrolysates obtained by papaya juice (HPJ 4 h), cantaloupe juice (HCJ 4 h), and papain (Hpapain 4 h), and unhydrolysed mixture of SPI with papaya juice (HPJ 0 h), cantaloupe juice (HCJ 0 h), and papain (Hpapain 0 h) for the ABTS•+-scavenging, •OH-scavenging, and tyrosine inhibitory capacities.
SPI hydrolysates by PJ, CJ, and papain in our study displayed more effective free radical-scavenging capacity than unprocessed SPI, which was similar to a previous study using papain and bromelain [Xu et al., 2023]. The improved antioxidant potential of hydrolysates can be attributed to the release of bioactive peptides during hydrolysis. The structural characteristics of these peptides, such as their molecular weight, amino acid composition, sequence, hydrophobicity, and secondary structure, determine their antioxidant potential [Zou et al., 2016]. For instance, C terminal Trp or Tyr-containing tripeptides from two libraries generated based on the sequence of the antioxidant peptides in the soy protein hydrolysates were found to exert strong radical-scavenging activities [Saito et al., 2003]. In another study, low molecular-weight fractions of soy protein hydrolysate had stronger radical scavenging effects than the higher molecular-weight fractions [Abu-Salem et al., 2013].
Wound-healing capacity of soy protein hydrolysates
The wound-healing property of peptides isolated from protein hydrolysates in previous studies [Gomes et al., 2017; Song et al., 2019], inspired us to examine this activity of the SPI hydrolysates obtained by PJ, CJ, and papain. Here, in vitro scratch assays on human fibroblasts were employed for the assessment of wound-healing property. Wound recovery was about 30% after 12 h and 50% after 24 h of treatment in the water-treated control, indicating the occurrence of self-wound-healing activity (Figure 4). SPI and Hpapain did not accelerate the healing process after 12 and 24 h, since similar percentages of wound closure were observed in the samples treated with SPI, Hpapain and water control. The same effects were also observed with HPJ 0 h and HCJ 0 h. On the contrary, HPJ 4 h and HCJ 4 h significantly ameliorated wound recovery in comparison with the other samples. Noticeably, at 24 h, wounds supplemented with HPJ 4 h and HCJ 4 h were closed by more than 70 and 90%, respectively. These results suggested that the wound-healing efficiency of SPI in the human fibroblast model was improved by the treatment with PJ and CJ but not with papain.
Figure 4
In vitro wound-healing activity of soy protein isolate (SPI), hydrolysates obtained by papaya juice (HPJ 4 h), cantaloupe juice (HCJ 4 h), and papain (Hpapain 4 h), and unhydrolysed mixture of SPI with papaya juice (HPJ 0 h), cantaloupe juice (HCJ 0 h), and papain (Hpapain 0 h). (A) Images of wound closure in human dermal fibroblasts treated with water (H2O, negative control) and samples at 0, 12, and 24 h. SPI hydrolysates were prepared at the juice to substrate ratio of 2.5:2 (v/w) or the papain to substrate ratio of 0.625:2 (w/w), temperature of 55°C, and reaction time of 4 h. (B) Quantification of wound closure (%) at 12 and 24 h. Each data bar is presented as mean and standard deviation of three independent experiments. Different letters above bars indicated significant differences among samples (p≤0.05).
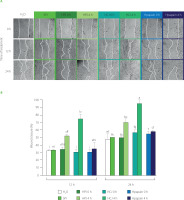
The distinctive composition of bioactive peptides in the SPI hydrolysates by fruit juices and papain might cause the difference in their wound-healing properties as peptides are capable of recovering wounds by influencing the inflammation, epithelialization, tissue granulation and remodeling steps [Gomes et al., 2017; Wang et al., 2022]. A recent study has shown that peptides prepared from the hydrolysis of soy protein with four commercial proteases promoted skin repair in rats through suppressing the excessive levels of factors in the inflammation step [Zhang et al., 2020a]. It would be interesting to investigate if peptides generated by the hydrolysis by PJ and CJ act via the inflammatory pathway or other mechanisms in a further study.
Tyrosinase inhibitory activity of soy protein hydrolysates
Natural anti-tyrosinase agents are of great interests in recent years as they can be excellent sources for skin whitening [Zhao et al., 2023]. Protein hydrolysates containing bioactive peptides have been shown to display outstanding anti-tyrosinase activities, implying their potential in the whitening area [Hu et al., 2022; Upata et al., 2022; Zhao et al., 2023]. Therefore, our study assessed this property in SPI hydrolysates obtained by PJ, CJ, and papain although it has not been described for soy protein hydrolysates so far.
Our data showed that HPJ 4 h, HCJ 4 h, and Hpapain 4 h inhibited the activity of tyrosinase more strongly than unhydrolysed SPI and respective HPJ 0 h, HCJ 0 h, and Hpapain 0 h (Figure 5). At the SPI concentration of 60 μg/mL, unhydrolysed SPI and Hpapain 0 h displayed about 15% of inhibition of tyrosinase activity whereas the percentages of inhibition were nearly 30 and 35% for HPJ 0 h and HCJ 0 h, respectively. The inhibitory effect on enzyme activity was remarkably increased in HPJ 4 h and HCJ 4 h (75% of inhibition) and moderately improved in Hpapain 4 h (35% of inhibition, respectively). Consistently, the IC50 values of HPJ 4 h (32.07 μg/mL), HCJ 4 h (30.49 μg/mL), and Hpapain 4 h (117.80 μg/mL) were lower than those of unhydrolysed SPI (>300 μg/mL), respective HPJ 0 h (116.40 μg/mL), HCJ 0 h (150.31 μg/mL), and Hpapain 0 h (>300 μg/mL) (Table 1). It is also worth mentioning that HPJ 0 h and HCJ 0 h showed stronger tyrosinase inhibitory activity than unhydrolysed SPI, suggesting that papaya and cantaloupe juices possessed the ability to inhibit tyrosinase. These results are consistent with the anti-tyrosinase property of the extracts from papaya and cantaloupe fruits [Le et al., 2023; Shin et al., 2008]. Protein hydrolysates obtained by enzymes of plant origin have been analysed for their inhibition of tyrosinase activity. For example, the hydrolysates of calf fleshing by-products obtained by papain and bromelain exhibited inhibitory effects on tyrosinase activity [Tedeschi et al., 2021]. The improvement of anti-tyrosisnase activity in the protein hydrolysates is possibly contributed by peptides as products of the hydrolysis process [Hu et al., 2022]. As SPI hydrolysates achieved by PJ and CJ in our study revealed strong tyrosinase inhibitory abilities, it is of importance to isolate bioactive peptides or peptide fractions from these hydrolysates and examine their skin-whitening activity in in vivo models.
Figure 5
Tyrosinase inhibitory capacity of soy protein isolate (SPI), hydrolysates obtained by papaya juice (HPJ 4 h), cantaloupe juice (HCJ 4 h), and papain (Hpapain 4 h), and unhydrolysed mixture of SPI with papaya juice (HPJ 0 h), cantaloupe juice (HCJ 0 h), and papain (Hpapain 0 h). SPI hydrolysates were prepared at the juice to substrate ratio of 2.5:2 (v/w) or the papain to substrate ratio of 0.625:2 (w/w), temperature of 55°C, and reaction time of 4 h. Each data bar is presented as mean and standard deviation of three independent experiments.
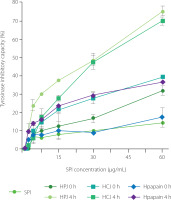
CONCLUSIONS
The present study results demonstrated the possibility of obtaining soy protein hydrolysates using papaya and cantaloupe juices with significantly higher ABTS•+ and •OH-scavenging, wound-healing, and anti-tyrosinase capacities compared to SPI. The commercial enzyme, papain, hydrolysing SPI at a relatively similar DH (about 11%) as PJ produced the hydrolysate with enhanced tyrosinase inhibitory and ABTS•+-scavenging capacities but insignificant wound-healing effect. The •OH-scavenging capacity of the hydrolysate obtained by papain was also improved to a lesser extent as compared to that of the hydrolysates produced with papaya and cantaloupe juices. Based on these results, papaya and cantaloupe juices offer great opportunities to produce SPI hydrolysates with perspective applications in healthcare foods, such as supplementary drinks.