INTRODUCTION
Extra virgin olive oil (EVOO) is widely known and consumed globally as a healthy source of dietary fat. It is produced from the fresh ripe olives and is considered as one of the most important agricultural products in the Mediterranean region [Muzammil et al., 2021]. The chemical composition of EVOO is highly dependent on various factors such as the cultivar of plant, agronomic practices involved in growing it, and geographical location of the crop [Ben Hmida et al., 2022]. EVOO is a staple food in the Mediterranean diet and is widely recognized for its health benefits [Jimenez-Lopez et al., 2020]. Olive oil is an essential ingredient in many traditional dishes, making it an important commodity in the food industry [Fernández-Lobato et al., 2022]. Its production and consumption are also an important part of the agricultural economy in many countries, including Tunisia [Fernández-Uclés et al., 2020]. The country is known for producing high-quality EVOO, with a unique flavor and chemical profile. Monovarietal EVOOs, which are produced from a single cultivar of olive, are gaining in popularity [Hlima et al., 2017]. In the Béja region of Tunisia, the Chemlali and Chétoui varieties were widely used to produce monovarietal EVOOs. Monovarietal EVOOs are highly prized for their unique taste and aroma, and considered to be among the best-quality EVOOs produced in Tunisia [Lechhab et al., 2022]. Understanding the chemical composition of these oils allows for the evaluation of their quality and identification of the factors contributing to their unique nutritional properties.
Analyzing the composition of monovarietal olive oil is important for several reasons. Firstly, it allows for the characterization and discrimination of different olive oil varieties [Aparicio & Luna, 2002; Kyçyk et al., 2016]. This is useful for both consumers and producers, as it helps to ensure the authenticity and quality of the oil. Secondly, analyzing the composition of monovarietal olive oil can provide valuable information for nutritional studies [Uncu & Ozen, 2016]. The health benefits of olive oil are well-known, and understanding the specific composition of different varieties can help to further explore these benefits. Additionally, knowledge about the composition of monovarietal olive oil can be useful in olive breeding projects [Monasterio et al., 2013]. For example, by identifying the specific sterol fraction of different varieties, breeders can select parents for new cultivars with improved characteristics [León et al., 2011]. Overall, analyzing the composition of monovarietal olive oil is essential for quality control, nutritional research, and the development of new olive varieties.
This study aimed to investigate the chemical composition variations in Chétoui monovarietal olive oils across Northern Tunisia’s Béja region. By conducting analysis of oils from thirteen mills, this study sought to uncover notable regional differences in essential chemical parameters, including free acidity; peroxide values; total phenolic content; and contents of chlorophylls, carotenoids, and tocopherols. Additionally, this research assessed the antioxidant potential of these olive oils, shedding light on the varying abilities of the polar and lipidic fractions to scavenge DPPH radicals, along with the fatty acid and triacylglycerol profiles. Ultimately, the findings emphasize the substantial impact of the collection region on the quality and nutritional attributes of Chétoui monovarietal olive oil, offering valuable insights for both scientific exploration and the olive oil industry.
MATERIALS AND METHODS
Chemicals
Reagents and standards used to perform spectrophotometric and titration analyses were purchased from Fluka (Buchs, Switzerland), Sigma-Aldrich (Saint Louis, MO, USA) or Merck (Darmstadt, Germany). The triacylglycerol standards, including trilinolein (LLL), triolein (OOO), tripalmitin (PPP), tristearin (SSS), trilinolenin (LnLnLn), and tripalmitolein (PoPoPo), each with a purity exceeding 98%, were acquired from Sigma-Aldrich (Saint Louis, MO, USA). We employed fatty acid methyl ester (F.A.M.E.) mix (CRM18918) from Supelco (Bellefonte, PA, USA) and α-tocopherol, β-tocopherol, and γ-tocopherol from Fluka (Buchs, Switzerland). All the solvents used for chromatographic methods were of the high performance liquid chromatography (HPLC) grade. n-Hexane, diethyl ether, acetone, and acetonitrile were provided by Panreac (Barcelona, Spain).
Sampling of Chétoui olive oils
Thirteen extra virgin olive oils (3×1,000 mL for each oil) of Chétoui olive variety from selected mills in the Béja region of Tunisia were used for the study. Three mills were located in Dogga (Dogga I, Dogga II and Dogga III), two mills each in Medjez El-Bab (Medjez El-Bab I and Medjez El-Bab II), Testour (Testour I and Testour II) and Nefza (Nefza I and Nefza II), and one mill each in Slouguia, Teboursouk, Tibar, and Amdoun (Figure 1). The Chétoui olives were harvested at the optimal stage of ripeness, and the farm operators followed proper grove management and oil processing practices. The olive oils were extracted by a continuous technological plant provided by three-phase centrifugal system. The fresh oils were stored in amber glass bottles at 4°C in the dark until analysis.
Determination of basic indicators of the virgin olive oil quality
Determination of free acidity
The free acidity was determined according the method recommended by the International Olive Council [IOOC, 2017b]. It was performed by dissolving 2 g of olive oil in 50 mL of a neutralized solvent mix (95% ethanol and diethyl ether, 50:50, v/v) in an Erlenmeyer flask and titrating the mixture with a 0.1 M ethanolic solution of potassium hydroxide in the presence of phenolphthalein until a lasting pink coloration appeared for at least 15 s. The free acidity was calculated as a percentage of oleic acid by weight (% oleic acid, w/w) using Formula (1).
where: V, volume (mL) of the KOH solution used, C, concentration (M) of the KOH solution used, M, molar mass of oleic acid (282 g/mol), and m, mass (g) of the sample taken.
Determination of peroxide value
The peroxide value of the examined oils was determined following the IOOC method [IOOC, 2017c]. This involved dissolving 1 g of olive oil in 10 mL of chloroform, 15 mL of glacial acetic acid, and 1 mL of potassium iodide (0.01 N). The mixture was stirred and kept in the dark for 5 min. Subsequently, 75 mL of distilled water and 1 mL of a starch indicator solution (resulting in a violet color) were added. The resulting solution was titrated with a solution of sodium thiosulfate (0.01 N) while vigorously stirring until a color change occurred, transitioning to a transparent color.
The peroxide value, expressed in milliequivalents of active oxygen per kilogram of oil, was calculated using Formula (2):
where: VE, volume of the sodium thiosulfate solution used for the mixture with test sample (mL), V0, volume of the sodium thiosulfate solution used for the blank (mL), and m, mass (g) of the sample taken.Ultraviolet spectrophotometry analysis
Specific extinction coefficients (K232 and K270) were determined following the method adopted by the IOOC [2019]. A 1% oil solution in cyclohexane was prepared (0.1g of oil in 10 mL of cyclohexane), and absorbances were measured at 232 and 270 nm. K232 and K270 were calculated using Formula (3).
where: Kλ, specific extinction coefficient at wavelength λ, Eλ, measured absorbance at wavelength λ, C, concentration of the solution (g/100 mL), and S, cuvette thickness (cm).Absorbance was measured at wavelengths 266 and 274 nm to calculate the change in specific extinction coefficients (∆K), expressed as in Formula (4):
Preparation of poplar and non-polar olive oil fractions
The separation of polar and non-polar oil fractions was performed according to procedure described by Kalantzakis et al. [2006]. This involved dissolving 2.5 g of each oil in 5 mL of n-hexane and then extracting it with a mixture of 5 mL of methanol and water (60:40, v/v) using a mechanical shaker (Vortex) to ensure thorough mixing. The mixture was than centrifuged at 1,350×g for 10 min to separate the non-polar fraction being obtained by evaporating the n-hexane from the upper layer of the supernatant and the polar fraction being collected as is.
Determination of total phenolic content of the extra virgin olive oils
The total phenolic content of the EVOOs was determined through colorimetric analysis using the Folin-Ciocalteu reagent, following the procedures outlined by Psomiadou & Tsimidou [2002]. The total phenolic content was measured in the polar oil fraction. A 13-µL aliquot of appropriately diluted fraction was placed in a tube in the presence of 50 µL of distilled water and 13 µL of the Folin-Ciocalteu reagent. After thorough agitation and a 3-min rest, 125 µL of a 7% Na2CO3 solution was added, and the mixture was adjusted with 100 µL of distilled water. The tube was then left to stand at room temperature and in the dark for 90 min, after which the absorbance was measured at 760 nm using a Cary 60 UV–Vis spectrophotometer (Agilent Technologies, Santa Clara, CA, USA). Results were reported as the equivalent of caffeic acid (mg CA/kg oil). Four replicates were performed for each sample.
Determination of chlorophyll and carotenoid contents of the extra virgin olive oils
The contents of carotenoids and chlorophylls of EVOOs were determined using a spectrophotometric method based on the work of Minguez-Mosquera et al. [1991], with measuring the absorbance at wavelengths of 470 nm and 670 nm, respectively. The values of the specific extinction coefficients used were 613 L/(g×cm) for pheophytin A as the main EVOO chlorophyll and 2,000 L/(g×cm) for lutein as the main EVOO carotenoid. The pigment contents were calculated by dividing the absorbance at the specified wavelength by the extinction coefficients of a reference compound, spectrophotometer cell thickness (1 cm), and a factor of 100. The result was expressed in mg of pheophytin A for chlorophylls and lutein for carotenoids per kg of oil. Four replicates were performed for each sample.
Determination of tocopherol composition of the extra virgin olive oils
The tocopherol composition was determined according to Manai-Djebali et al. [2012]. The analysis involved dissolving the oil in n-hexane and analyzing the solution using an Agilent 1200 HPLC with a silica gel Lichrosorb Si-60, 5 μm particle size, length (L) × inner diameter (I.D.) of 25 cm × 4 mm column (Agilent Technologies, Santa Clara, CA, USA). The elution was accomplished using a mixture of n-hexane and 2-propanol (99:1, v/v), and the flow rate was set at 1 mL/min. A fluorescence detector was used for detection, with the excitation and emission wavelengths set at 290 and 330 nm, respectively. The content of individual tocopherols was reported as mg per kg of oil. Identification was accomplished by comparing the retention times of α-, β-, and γ-tocopherols. The quantification was performed using a calibration curve for the three tocopherols, and the coefficients of determination (R2) for α-, β-, and γ-tocopherols were 0.989, 0.999, and 0.991, respectively. Four replicates were performed for each sample.
Determination of fatty acid composition of the extra virgin olive oils
Methyl esters of EVOO fatty acids (FAs) were prepared by vigorous shaking of the oils in n-hexane with 0.2 mL of a 2 M methanolic potassium hydroxide solution [IOOC, 2017a]. The gas chromatography separation was performed using an Agilent 6890N gas chromatograph (Agilent Technologies, Wilmington, DE, USA) equipped with a flame ion detector (FID) and an HP-1 (polydimethylsiloxane) fused-silica capillary column with an L of 50 m, an I.D. of 0.2 mm and a film thickness of 0.33 mm. The carrier gas was helium, delivered with a constant flow rate of 1 mL/min, and an oven temperature was programmed from 60 to 250°C at a rate of 2°C/min, and then held isothermal for 20 min. The FID temperature was set at 250°C. The identification of FAs was performed by comparing the specific retention time of each compound with those of a fatty acid methyl ester standard (F.A.M.E. Mix, CRM18918-Supelco, Bellefonte, PA, USA). Four replicates were performed for each sample, the results were expressed in area %.
Determination of triacylglycerol composition of the extra virgin olive oils
According to Commission Implementing Regulation (EU) Amending Regulation (EEC) No. 2569/91 [2016], the analysis of triacylglycerols (TAGs) was performed by dissolving 0.12 g of olive oil in 0.5 mL of n-hexane and then purifying the triacylglycerol fraction using solid-phase extraction (SPE) with a Silica column and a mixture of n-hexane and diethyl ether (87:13, v/v). After evaporation, the purified triacylglycerols were dissolved in 2 mL of acetone and analyzed using an Agilent 1200 HPLC Series (Agilent Technology, Palo Alto, CA, USA) equipped with a refractometric detector and a LiChrospher RP-18, 5 μm particle size, L × I.D. of 25 cm × 4.6 mm column (Merck, Darmstadt, Germany). The elution solvent was a mixture of acetone and acetonitrile (50:50, v/v), and the flow rate was set at 1.2 mL/min. Triacylglycerols, including LLL, OOO, PPP, SSS, LnLnLn, and PoPoPo, were used as standards (Sigma-Aldrich, St Louis, MO, USA). To determine TAGs, the retention times were graphed by comparing them to reference chromatograms from soybean oil, a 30:70 (w/w) mixture of soybean oil and olive oil, and pure olive oil, following the methodology outlined in IOOC [2017d]. It was presumed that the total area of peaks representing different TAGs added up to 100%, and the proportional distribution of each TAG was subsequently computed.
Determination of radical scavenging activity of the extra virgin olive oils
The ability of the of EVOO polar and non-polar fractions to scavenge the DPPH radical was determined according to Kalantzakis et al. [2006] by adding 250 µL of each oil fraction in ethyl acetate (10%, w/v) to 1 mL of a freshly prepared DPPH radical solution (10 mM in ethyl acetate) in a 2-mL test tube. The mixture was shaken vigorously for 10 s using a Vortex mixer and allowed to stand in the dark for 30 min until a steady state was reached. Its absorbance was then measured at 515 nm and compared to that of a solution only with the DPPH radical (without sample). The DPPH radical scavenging activity of olive oil fractions was expressed in % DPPH radical inhibition.
Statistical analysis
The data analysis and hierarchical cluster analysis (which involved grouping olive oil provenances based on the studied parameters) were performed using JMP 14 software (SAS Institute Inc., Cary, NC, USA). Statistical computations were conducted using one-way analysis of variance (ANOVA) followed by the Newman– Keuls multiple comparison test. A significance level of p<0.05 was chosen, indicating a common threshold used to determine statistical significance.
RESULTS AND DISCUSSION
Basic indicators of the extra virgin olive oil quality
The quality assessment of EVOOs from the 13 locations showed significant varying levels of free acidity, peroxide value, K232, K270 and ΔK (Figure 2). The free acidity values offer insights into the quality and freshness of the olive oils produced. Notably, the results revealed variations in free acidity among olive oils from different mills. For instance, oils from Nefza II and Slouguia demonstrated the lowest free acidity, with 0.57 and 0.58% oleic acid (w/w), respectively. These findings suggest the possibility of higher quality and lower oxidation in these oils. Conversely, Amdoun and Tibar exhibited the highest free acidity, reaching 0.73 and 0.72% oleic acid (w/w), respectively. The acidity level should be less than 0.8% in virgin olive oil and less than 2% in refined olive oil [Jimenez-Lopez et al., 2020]. High levels of acidity can affect the taste, odor, and stability of the oil [Issaoui et al., 2011].
High peroxide values indicate that the oil has been exposed to light, heat, and/or oxygen, which can lead to a rancid taste and odor. The EVOO with the highest peroxide value was from Dogga II, with a mean value of 14.00 meq O2/kg, while the lowest peroxide value was found in oils from Slouguia and Dogga I, with a mean value of 8.17 meq O2/kg and 7.33 meq O2/kg, respectively (Figure 2). The specific extinction coefficients (K232, K270 and ΔK) are other indicators of oil oxidation, providing information about its quality and preservation state. K232 indicates the formation of conjugated diene hydroperoxides, primary oxidation products, in the oil. K270 is associated with the formation of secondary products of oxidation, such as aldehydes and ketones. The highest K232 mean value (2.47) was recorded in EVOO from Amdoun, while the lowest value (1.86) was determined in oil from Testour II (Figure 2). The highest K270 values were assayed in EVOO from Testour I and Testour II, with mean values of 0.21 and 0.22, respectively. Additionally, it is worth noting that the olive oils from regions of Tibar, Teboursouk, Nefza I, Nefza II, Slouguia, Medjez El-Bab I, Medjez El-Bab II, and Dogga I exhibited statistically similar (p≥0.05) K270 values, ranging from 0.19 to 0.21. These oils share comparable chemical characteristics in this specific measure. The lowest K270 was recorded in oils from Amdoun and Dogga III, with a mean value of 0.15 for both oils. The highest ΔK value was found for oil from Medjez El-Bab II, with a mean value of 0.0065, while the lowest value was determined for EVOO from Tibar, with a mean value of 0.0012.
Figure 2
Free acidity (A), peroxide value (B), and extinction coefficients including K232 (C), K270 (D) and ΔK (E) of the Chétoui extra virgin olive oils from selected mills in the Béja region in Tunisia. Data are expressed as box plots for four replicates. Means in the same box bearing different letters are significantly different at p<0.05.
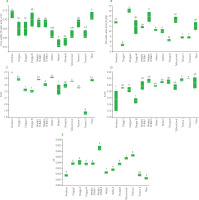
The quality of the monovarietal EVOOs, evaluated based on several key indicators, met the standards set for this type of products. All values fell within the standards set for “extra virgin olive oil” as defined by the IOOC [IOOC, 2021], which recommend acidity of less than or equal to 0.8%, peroxide value of less than or equal to 20 meq O2/kg, K270 of less than or equal to 0.22, K232 of less than or equal to 2.5, and ΔK of less than or equal to 0.01. The quality of the oil is considered higher if the values of these parameters are lower, as this usually indicates that the olives used were fresh and healthy, harvested at the optimal ripening stage, and processed immediately without storage. The results of our study align with those of previous research conducted on the oils of Chétoui variety in Tunisia [Ben Hmida et al., 2022]. This consistency supports the validity of our findings and adds to the existing body of knowledge on the oils of Chétoui variety in Tunisia.
Content of total phenolics
Phenolics are a class of compounds found in olive oil that have antioxidant properties [Bendini et al., 2007]. High content of phenolics is associated with a high oil stability and a low level of oxidation and degradation [El Yamani et al., 2019]. The highest total phenolic content was found in EVOO from Testour II, with a mean value of 1298.60 mg CA/kg, while the lowest value was determined in oils from Amdoun with a mean value of 906.53 mg CA/kg (Figure 3). These differences were found to be statistically significant (p<0.05), highlighting the substantial variations in total phenolic content among these regions. The contents of phenolics in olive oil can vary depending not only on the plant cultivar and the region of its production, but also on agricultural practices and the fruit processing method [Ben Youssef et al., 2012; Lechhab et al., 2022]. The total phenolic content was found to be influenced by the irrigation regime, with rain-fed Chétoui olives showing higher levels of total phenolics than those grown with irrigation [Ben Youssef et al., 2010; Haddada et al., 2007].
Content of chlorophylls and carotenoids
Figure 3 displays the variation of chlorophyll and carotenoid contents in EVOOs from different locations in Béja region. The chlorophyll content was found to vary greatly across region, with oils from Dogga II and Amdoun having the highest content (7.85 and 7.54 mg/kg, respectively) and in those from Slouguia having the lowest one (2.03 mg/kg). This variation in chlorophyll content was also reported in previous studies by Ben Youssef et al. [2010], who found that the chlorophyll content in Chetoui oils from olives at different stages of maturity varied from 9.90 to 3.35 mg/kg for a ripening index of 1.18 to 5.47. Guerfel et al. [2009] also found that the chlorophyll content varied based on the growing region, with values ranging from 2.5 to 9.8 mg/kg. The carotenoid content in the olive oils also exhibited significant variation, with samples from Amdoun and Dogga II displaying the highest content (3.92 and 3.88 mg/kg, respectively), compared to the lowest value noted for Slouguia (1.28 mg/kg). These differences were found to be statistically significant. Ben Youssef et al. [2010] reported the carotenoid content in Chetoui oils to vary from 4.34 to 1.49 mg/kg for a ripening index of 1.1 to 5.47. Guerfel et al. [2009] also found variation in carotenoid content based on the cultivation region.
Figure 3
Total phenolic content (A), carotenoid content (B) and chlorophyll content (C) of the Chétoui extra virgin olive oils from selected mills in the Béja region in Tunisia. Data are expressed as box plots for four replicates. Means in the same box bearing different letters are significantly different at p<0.05. CA, caffeic acid equivalent.
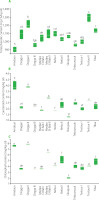
Tocopherol composition
Tocopherol is an important factor in determining the quality of olive oil. Tocopherols are a family of vitamin E compounds that are found in many vegetable oils, including olive oil [Beltrán et al., 2010]. They play an important role in olive oil quality because they serve as natural antioxidants, which help to prevent oxidation and preserve the freshness of the oil. In particular, α-tocopherol is the most abundant in olive oil and has the highest antioxidant activity [Blekas et al., 1995]. The content of α-tocopherol in olive oil is often used as an indicator of its overall freshness and quality. Oils with higher content of α-tocopherol are less likely to have undergone oxidative spoilage and are therefore less likely to develop off-flavors or odors [Deiana et al., 2002].
The contents of α-, β-, and γ-tocopherols of olive oils from different mills located in the Béja region are shown in Figure 4. The highest total tocopherol content was found in oils from Testour II (416.79 mg/kg), Medjez El-Bab II (410.80 mg/kg), and Testour I (400.98 mg/kg). On the other hand, the lowest total tocopherol content was found in EVOOs from Tibar (282.88 mg/kg) and Nefza II (303.89 mg/kg), followed by Nefza I (337.34 mg/kg), and Amdoun (342.03 mg/kg). When comparing the α-, β-, and γ-tocopherol contents, as expected, the highest values were found for α-tocopherols. Similarly, to the total tocopherol content, the highest α-tocopherol content was found in the samples from Medjez El-Bab II (394.00 mg/kg) and Testour II (395.51 mg/kg), whereas the lowest in the oils from the mills in Tibar (263.83 mg/kg) and Nefza II (286.72 mg/kg). Oil from Testour II mill exhibited the highest β-tocopherol content at 7.61 mg/kg, and this value was significantly higher (p<0.05) compared to these for oil from mills in Dogga I (with the lowest β-tocopherol content at 5.30 mg/kg,) Nefza II, Nefza I, Dogga III, Amdoun, and Slouguia. Upon conducting ANOVA of all the oil samples, there were no significant (p≥0.05) differences in γ-tocopherol content, which ranged from 9.93 mg/kg in Medjez El-Bab II to 13.67 mg/kg in Testour II. These results suggest that the level of γ-tocopherol does not differentiate monovarietal extra virgin olive oils across the mills and regions.
Figure 4
Content of α-tocopherol (α-Toc) (A), β-tocopherol (β-Toc) (B) and γ-tocopherol (γ-Toc) (C) of the Chétoui extra virgin olive oils from selected mills in the Béja region in Tunisia. Data are expressed as box plots for four replicates. Means in the same box bearing different letters are significantly different at p<0.05.
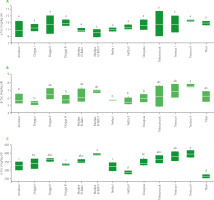
The tocopherol content of olive oil has been extensively studied and our findings align with the following study. BenTemime et al. [2006] found that the total tocopherol content was influenced by the production area, ranging from 341.40 mg/kg (Lakhouet) to 405.65 mg/kg (Amdoun). In the cited work, similarly to our study, the analysis of the tocopherols using HPLC showed the presence of their three forms (α-, β-, and γ-tocopherols) with α-tocopherol being the main isomer, and the variation in the total tocopherol contents in oils, which was reflected by changes in α-tocopherol content (from 324.32 mg/kg of Lakhouet oil to 385.35 mg/kg of Amdoun oil); β-, and γ-tocopherols were less represented, with contents not exceeding 12 mg/kg. According to Hassine et al. [2015], the α-tocopherol content in Testour oil was 477.26 mg/kg and in Medjez El-Bab it was 382.26 mg/kg.
Fatty acid compositions
Fatty acids played an important role in the human diet and had a variety of health effects. They are divided into saturated fatty acids (SFAs) and unsaturated fatty acids (UFA), including monounsaturated fatty acids (MUFAs), and polyunsaturated fatty acids (PUFAs). Previous studies showed that SFAs were implicated in adverse health effects, such as an increased risk of cardiovascular disease, while UFAs were linked to positive health effects, such as reducing the risk of cardiovascular disease [Islam et al., 2019]. The ratio of MUFAs to PUFAs was also considered an indicator of the quality of fatty acid content [Chen & Liu, 2020].
The fatty acid composition of EVOOs from different mills located in the Béja region is shown in Table 1. It was observed that the SFA content was statistically consistent (p≥0.05) across the region, except for the oil from Tibar, which displayed the lowest SFA content at 14.55% of total fatty acids. When it comes to UFAs, there were no statistically significant (p≥0.05) differences among the olive oil samples tested, ranging from 83.42% to 85.45%. The MUFA content was also relatively uniform across the region, except for oil from Tibar, which stood out with the highest value of 72.04%. Notably, the PUFA content exhibited significant variability, with the highest value found in the oil from the mill in Amdoun at 18.29% and statistically similar (p≥0.05) in several other oils, including those from Medjez El-Bab I, Medjez El-Bab II, Dogga III, Dogga I, Slouguia, and Teboursouk ranging from 16.99% to 18.06%. Additionally, the C18:1/C18:2 ratio exhibited significant (p<0.05) differences, with the highest value recorded in the oil from Tibar (5.62) and the lowest in the oils from Amdoun (3.74), Medjez El-Bab I (3.81) and Medjez El-Bab II (3.96). The variation in the amount of fatty acids in olive oils from the Chétoui cultivar (Table 1) was likely due to a combination of genetic factors and environmental conditions during fruit growth and maturity. The fatty acid content in the oil was also influenced by the ripeness of the fruit. If harvesting was delayed, the levels of unsaturated fatty acids, especially linoleic acid, tended to increase while the levels of palmitic acid to decrease [Ben-Temime et al., 2006]. The results obtained in our study are in line with previous research conducted by Ben-Temime et al. [2006], Ben Youssef et al. [2010; 2012], and Yahia et al. [2012]. This consistency in findings across various studies reinforces the robustness of our observations and suggests that the patterns in fatty acid composition in olive oils from different regions, especially the distinct characteristics of Tibar and Amdoun, are well-documented and established in the scientific literature.
Table 1
Fatty acid composition (% total fatty acids) of the Chétoui extra virgin olive oils from selected mills in the Béja region in Tunisia.
Triacylglycerol composition
Triacylglycerols (TAGs) are the main constituents of olive oil. TAGs consist of a glycerol molecule attached to three fatty acid chains and are responsible for the oil’s physical and chemical properties, such as viscosity, stability, and oxidative behavior [Sánchez & Harwood, 2002]. The fatty acid composition of TAGs is one of the most important factors that affects olive oil quality. It influences the oil’s flavor, aroma, and stability, as well as health benefits [Harwood & Yaqoob, 2002]. For example, olive oil with a high MUFA content, such as oleic acid, is considered to have a better flavor and aroma profile, as well as a longer shelf life, compared to oils with a high PUFA content [Garcia-Oliveira et al., 2021]. The percentage composition of triacylglycerols detected in Chétoui extra virgin olive oil samples from different locations in Béja region in Tunisia is shown in Table 2. The triacylglycerols included oleodilinolein (LLO), dioleolinolenin (OLnO), dioleolinolein (OLO), palmitolinoleoolein + stearodilinolein (PLO+SLL), dipalmitolinolein (PPL), triolein (OOO), palmitodiolein (POO), dipalmitooleotin (PPO), dioleostearin (SOO), and linoleodistearin + palmitooleostearin (SLS+POS). The data in Table 2 reveals significant disparities in the composition of TAGs in monovarietal olive oils. Notably, the LLO content ranged from 3.56% in the oil from Tibar to 6.36% and 6.92% in the oils from Dogga III and Dogga II, respectively, and these differences could be potentially attributed to variances in environmental conditions for growing olive trees. In the case of OLnO, the highest contents at 5.70% and 5.62% were found in the oils from Dogga II and Dogga III, respectively, while the oil from Tibar presented the lowest at 1.95%. Furthermore, mills located in Amdoun, Testour I, Medjez El-Bab I and Medjez El-Bab II (oils with a peak OLO percentage of 18.02–18.62%) may produce olive oils with enhanced shelf stability, compared to Dogga II and III mills producing oils with the lowest OLO content at 14.31–14.45%. The data indicates that the percentage content of POO was minimal in Amdoun (20.95%) and the highest in Dogga III (22.99%) olive oil, and there were no significant (p≥0.05) differences among these regions in terms of POO content. For SOO content, there was a significant variability among the regions, with values ranging from 2.47% in Dogga III to 4.79% in Nefza I samples. In general, the triacylglycerol composition of the different olive oil samples showed a wide range of variations, reflecting the diversity of the different regions in terms of climate, soil, and cultivation practices. It was therefore an important factor to consider when evaluating the quality of olive oil [Haddada et al., 2007].
Table 2
The triacylglycerol composition (% total triacylglycerols) of the Chétoui extra virgin olive oils from selected mills in the Béja region in Tunisia.
[i] Data are expressed as average of four replicates ± standard deviation. The triacylglycerol names were abbreviated by means of three letters corresponding to the fatty acid bound to the glycerol backbone. In alphabetic order: L, linoleic acid (C18:2); Ln, linolenic acid (C18:3); O, oleic acid (C18:1); P, palmitic acid (C16:0); and S, stearic acid (C18:0). Values with different lowercase letters in superscript were significantly different at p<0.05
DPPH radical scavenging activity of polar and non-polar oil fractions
The results of DPPH radical scavenging activity assay of polar and non-polar fractions of EVOOs taken from different locations are shown in Figure 5. The DPPH radical scavenging activity of the polar fraction was found to be relatively high, ranging from 93.26% for Testour II sample to 95.69% for Dogga III sample. On the other hand, the non-polar fraction showed a lower DPPH radical scavenging activity, ranging from 44.80% to 74.52%, with oils from Medjez El-Bab I and Dogga II having the highest percentage. There was a significant difference in the DPPH radical scavenging activity between the polar and non-polar fractions for all oils, with the polar fraction showing a much higher percentage of DPPH inhibition. This finding is consistent with other studies that have evaluated the antioxidant properties of Chétoui olive oil. For example, a study by Ben-Temime et al. [2006] reported that the polar fraction of Chétoui olive oil had a higher total phenolic content and antioxidant activity than the lipid fraction. Another study by Nakbi et al. [2010] found that the phenolic compounds in Chétoui olive oil contributed to its antioxidant activity. Additionally, a study by Issaoui et al. [2015] reported that the antioxidant activity of Chétoui olive oil was influenced by several factors, including the maturity stage of the olives and the oil extraction method [Damak et al., 2008].
Figure 5
DPPH radical scavenging activity of the polar fraction (RSA-PF) (A) and non-polar fraction (RSA-NPF) (B) of the Chétoui extra virgin olive oils from selected mills in the Béja region in Tunisia. Data are expressed as box plots for four replicates. Means in the same box bearing different letters are significantly different at p<0.05.
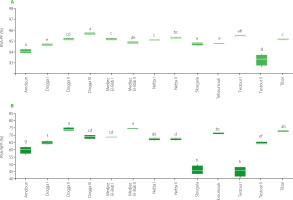
Hierarchical clustering analysis of olive oils
After performing hierarchical clustering (Figure 6), it is evident that oils from mills of some regions of olive oil production share common characteristics while others differ significantly.
Figure 6
Dendrogram of hierarchical clustering analysis of the Chétoui extra virgin olive oils from selected mills in the Béja region in Tunisia according to their physicochemical properties.
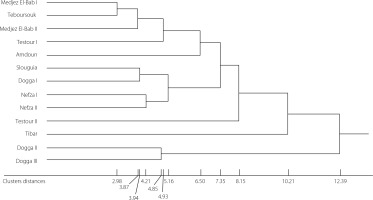
The regions that were most similar in terms of the characteristics of the olive oil collected were Medjez El-Bab I and Teboursouk (cluster distance of 2.98), Medjez El-Bab I and Medjez El-Bab II (distance of 3.87), Slouguia and Dogga I (distance of 3.94), Nefza I and Nefza II (distance of 4.21), Dogga II and Dogga III (distance of 4.85), Medjez El-Bab I and Testour I (distance of 4.93), and finally Slouguia and Nefza I (distance of 5.17). On the other hand, the regions that are furthest apart were Medjez El-Bab I and Amdoun (cluster distance of 6.50), Medjez ElBab I and Slouguia (distance of 7.35), Medjez El-Bab I and Testour II (distance of 8.15), Medjez El-Bab I and Tibar (distance of 10.21), and finally Medjez El-Bab I and Dogga II (distance of 12.39). These regions had olive oils with characteristics that differed significantly from the other regions. This classification is useful for understanding the differences and similarities between olive oils collected throughout the Béja region and can help identify appropriate strategies to improve olive oil production in this region.
CONCLUSIONS
In conclusion, our investigation of monovarietal extra virgin olive oils from Tunisian mills in the Béja region revealed significant disparities in key chemical parameters among the oils from 13 different mills. These findings underscore the remarkable diversity of Tunisian olive oils, strongly influenced by their geographical origins. Noteworthy, the oils from Testour II displayed the highest total phenolic content, while the oils from Amdoun and Dogga II exhibited high chlorophyll and carotenoid levels. The oil from Amdoun had lower tocopherol content compared to these from Testour II, Medjez El-Bab II, and Testour I mills. Fatty acid profiles were mostly similar across regions, except for the oil from Tibar with the lowest SFA content and highest MUFA content. Furthermore, substantial differences in PUFA content were observed, with the oil from Amdoun having the highest levels. Triacylglycerol compositions also reflected these regional distinctions. Lastly, our study revealed notably higher antioxidant activity in the polar fraction, especially in the olive oil from Dogga III. These results underscore the significant influence of geographical origin on the chemical composition and quality of Tunisian olive oils, emphasizing the importance of considering regional disparities in the evaluation and selection of olive oils. Additionally, they highlight the potential of these oils as natural sources of antioxidants.