INTRODUCTION
Red raspberry (Rubus idaeus L.) is a perennial crop of the Rosaceae family. The fruit is an aggregate of drupelets with a hollow centre. It is commonly cultivated in at least 30 countries in the world [Kalušević et al., 2016; Wang et al., 2009], including Italy. Specifically, it is cultivated in the soil or in pots in Piedmont, in the North-West of Italy. Raspberry cultivation in pots is gaining more relevance. First of all, this technique allows for better space and resource management: water is supplied directly to each plant, avoiding waste. In addition, the introduction of additives, minerals, or vitamins is more efficient in pots because all the nutritive substrate − that is to say potting soil or other plant growing support − is in direct contact with the plant roots. Changes in the raspberry production system have led to the use of pots, nutritive substrates, polytunnels and other protective structures, allowing a better administration of the microclimatic conditions and of the overall production system, also limiting the possible spread of diseases, pests, and possible contaminations from the environment. Moreover, the soil or other substrate used for the growth of the raspberry plant can affect berry characteristics, such as the quality and quantity of bioactive compounds or the fruit yield [Balawejder et al., 2023]. In addition, the market demands fresh produce all year long, a requirement which is not possible to meet with traditional open-field cultivation. Advantages from pot-grown raspberry cultivation also include the possibility of using the same area for many years, improving yields, fruit quality and size, and favouring a better organisation of working and picking activities [Balawejder et al., 2023; Evdokimenko et al., 2021; Linnemannstöns, 2020].
Berries are highly appreciated by consumers, who choose to consume them because of the noticeable content of secondary metabolites; they are actually sources of bioactive compounds such as phenolic compounds with antioxidant activity, mainly anthocyanins, which are a subgroup of flavonoids also responsible for the colour of this small berry fruit [Frías-Moreno et al., 2021; Gimeno et al., 2022; Kalušević et al., 2016; Toshima et al., 2021; Villamor et al., 2013]. For this reason, they have gained economic importance and their cultivation is more widespread every year [Nile & Park, 2014]. Most berries are deemed potentially beneficial for human health, even if many mechanisms and metabolic actions are not precisely known yet. The emphasis should be placed on attributing significant health-promoting properties to berry fruits, thereby elevating their status above the already acknowledged positive attributes of fruits and vegetables. Numerous studies already show anticancer, antimutagenic, anti-inflammatory, and antioxidant activity, with cardiovascular-protecting characteristics related to the berry consumption [Cardona et al., 2013; Paredes-López et al., 2010; Rasmussen et al., 2005]. Beattie et al. [2005] highlight that phytochemicals, as non-nutritive dietary components with broad bioactivity, often elicit health-promoting benefits in the human body. While their absence does not necessarily lead to deficiency conditions, they can potentially exert positive effects on cells as promoters of health, substances that combat diseases, and substances that prevent diseases.
The content of phytochemicals in raspberries depends on (1) intrinsic factors, such as the cultivar, (2) harvesting factors, such as the cultivation area and the environmental conditions, (3) storage temperature and postharvest management, and (4) treatments and processing [de Souza et al., 2014; Nile & Park, 2014]. The fragility and perishability of raspberries makes their preservation an issue. Due to their high water content and sugar composition, they are easily subjected to moulds, yeasts, and bacteria development [Kalušević et al., 2016; Wang et al., 2009]. In the coming years, the industry needs to confront the challenge of discovering novel approaches to maintain the nutritional and microbial integrity of these fruits. Additionally, efforts could be made to enhance the levels of polyphenols and other antioxidants, thus augmenting the value of the end product. An innovative method to accomplish this objective involves using ultraviolet (UV) rays, which belong to the section of the electromagnetic spectrum encompassing wavelengths between 100 and 400 nm. This can be further divided into three sections where UVA, UVB and UVC rays are identifiable. Specifically, the exposure to UV light in wavelength ranges of–280–315 nm (UVB) and 100–280 nm (UVC) has been studied as alternatives to heating treatments, such as pasteurization, and as elicitor treatments to increase the content of bioactive compounds in fruit and vegetables [Darré et al., 2022; Koutchma, 2014]. These ultraviolet rays, mainly UVC, are actually used industrially for the disinfection of water, air and surfaces, because their wavelengths alter the DNA of the microorganisms [Darré et al., 2022; Kebbi et al., 2020; Koutchma, 2014]. A low microbial contamination is an indication of good quality and safety for all products. Small berry fruits were responsible for outbreaks of foodborne diseases in both Europe and North America, and raspberries were specifically linked to 11 outbreaks between 1983 and 2013 [Xu & Wu, 2016]. The main pathogens involved were human noroviruses, including Salmonella, which has also been recognised as an issue by European Food Safety Authority (EFSA), and Escherichia coli O157:H7 [Butot et al., 2018]. The high water and sugar content in berries provide an ideal environment for the growth and proliferation of moulds, for example, Botrytis cinerea, an agent of grey mould and responsible for raspberry decay [Butot et al., 2018; Gimeno et al., 2022; Xu & Wu, 2016].
The most common source of UV rays up to now is constituted by mercury lamps, but some problems can occur with the disposal. Mercury is a dangerous and toxic element, and specific treatment for mercury-containing waste is necessary. Conversely, the light-emitting diode (LED) technology has many advantages and can also be considered for post-harvest treatment. LED lamps offer several advantages, including their affordability in terms of purchase, management, and maintenance. They are also safe for operators and environmentally friendly due to the absence of mercury and their long lifespan, which contributes to reduced environmental impact. LEDs can be adjusted to various wavelengths, do not pose significant heating issues, and provide immediate effectiveness upon being switched on [Chawla et al., 2021; Koutchma, 2014].
The application of UV radiation on red raspberries has not yet been investigated in depth; however, it has been discussed in a piece of research by Gimeno et al. [2022]. They showcased their study on utilizing UV treatment in conjunction with modified-atmosphere packaging (MAP) to regulate decay and improve quality attributes during the postharvest storage of raspberries. Results showed that the higher CO2 level is actually helpful in the storage of the fresh product because of reducing its metabolic activity. More vivid colour was achieved, indicating that the anthocyanins were better preserved; the overall decay of the berries was delayed; the loss of firmness was reduced thanks to the coupled effects of MAP and UVC; an increase in the level of flavonoids was also achieved. The best results to improve raspberry storage came from combining the modified atmosphere and light treatment [Gimeno et al., 2022].
The objective of this research was to develop a postharvest treatment method for red raspberries that would enhance their preservation and preserve their quality characteristics. This was achieved by employing UVC-LED radiation in both soil and pot cultivations. Specifically, the objective was to understand the effect of UVC light treatment on the quality of raspberries exposed to the rays and later stored at 4°C and 20°C for 72 h, highlighting potential dissimilarities between pot-grown and soil-grown fruits.
MATERIALS AND METHODS
Plant material
Red raspberries of the “Grandeur” cultivar were chosen for the experiments. This cultivar is characterised by berries with an average weight of 3.5–3.6 g, conical shape and intense red colour [Ackerman & Adams, 2009]. These berries were collected from a local producer from Cuneo, Piedmont (Italy), where the cultivation was managed in soil (S) and in pots (P). All plants of raspberry were grown under hail net. Chemical plant protection was not applied. Irrigation was applied using sprinklers as needed and a fertigation system was applied to guarantee water supply with a commercial fertigation recipe for raspberry. Plots were established by digging up soil and transferring it to 7-L pots. The floricanes were manually pruned out each year and fruits were manually harvested. For both soil-grown and pot-grown raspberries, a treated (TR) and a control (C) sample were considered and two storage temperatures (4°C and 20°C) tested. Raspberries were divided into polyethylene (PE) baskets, 6 for each experimental variant (S C 4, S TR 4, P C 4, P TR 4, S C 20, S TR 20, P C 20, P TR 20) and arranged as a single layer. Each basket contained 12 fruits.
Postharvest ultraviolet light treatment
The ultraviolet light treatment of raspberries was performed with a prototype instrument made for DISAFA by PRO.LUX S.r.l. (Druento, Turin, Italy), a company by MOVE2WEB S.r.l. (Turin, Italy). This instrument consists of a stainless-steel box with 9 tracks inside on which a stainless-steel tray can be placed. The LED plate is positioned on the internal top vault of the prototype; it consists of 20 LED lines (10 emitting UVB rays and 10 emitting UVC, which are switched on separately). Each line is completed by a blue-light LED which turns on when the diodes are functional. Our research activity required only the use of UVC-LED lamps. The treatment was carried out by positioning the baskets with the berries at level 2, 157 mm far from the LED plate. The samples were exposed to UV in a wavelength range between 270 nm and 285 nm and to a power of 14.17 W/m2 for 14 min, providing a total radiation dose of 11.90 kJ/m2. The UV-LED treatment was performed on 6 baskets.
Storage conditions
After the radiation treatment (TR), the samples were divided into 2 groups, the first stored in a climate-controlled room at 20°C and the second stored at 4°C for 72 h in a refrigerated room. Every sample was analysed at the starting time of the experiment, which is to say when received in the laboratory, prior to the UVC exposure (start), and after the preservation period. The samples treated with radiation were compared to non-treated samples, referred to as control (C). Control samples from soil and pot cultivation and for each storage condition were considered.
Weight loss determination
Each basket containing raspberries was weighed at start time (weightstart) and after the storage period (weightfinal) with a precision analytical scale (Kern & Sohn GmbH, Balingen, Germany), and weight loss was evaluated in percentage (WL%) according to the following formula (1):
Determination of colour parameters
The colour parameters of the red raspberries were evaluated on the surface of the fruit with a CR-400 colorimeter (Konica Minolta, Chiyoda, Japan) in CIELab tristimulus coordinate system: L* index of brightness ranging from 0 to 100, a* index showing colour turning from green to red and ranging from −120 to +120, and b* index showing colour turning from blue to yellow and ranging, again, from −120 to +120. An additional parameter considered was hue angle (h°), suggesting the shade of the colour. It was calculated according to the formula (2):
Data was processed to obtain the differences (∆) between the measurement at the start time and the data achieved after the 72-h storage. In this way, ∆L*, ∆a*, ∆b* and ∆h° were obtained.Texture profile analysis
Texture profile analysis (TPA) of raspberries was performed according to the method described by Giongo et al. [2019] with the Texture Analyzer instrument (Stable Micro System, Godalming, United Kingdom). The flat stainless-steel probe had a diameter of 75 mm, test speed was set at 300 mm/min, the application of the charge was 20% over the deformation, and the nominal trigger force was set to 2 g. Of all 7 parameters (hardness, adhesiveness, springiness, cohesiveness, gumminess, chewiness, resilience) obtained with the analysis of all 12 raspberries in the basket, only hardness, chewiness, and resilience were considered.
Total phenolic content, ferric reducing antioxidant power and total anthocyanin content determination
Fruit extracts were prepared for the subsequent quantification of phenolic compounds and ferric reducing antioxidant power (FRAP) of raspberries. A portion of 5.0 g of previously cut raspberries, which were randomly chosen among the 6 baskets prepared for each experimental variant, were added to 12.5 mL of extraction solvent (500 mL of methanol + 24 mL of deionised H2O + 1.4 mL of 12 M HCl). The preparation was set in the dark for 2 h, then homogenised with Ultra Turrax (Janke and Kunkel, IKA®-Labortechnik, Staufen, Germany) until it became smooth. Samples were then centrifuged at 2,500×g for 15 min. The supernatant was collected and used for the quantification of bioactive compounds. Following the method described by Pantelidis et al. [2007], total phenolic content (TPC) determination involved the use of Folin-Ciocâlteu’s reagent. To the mixture, 40 μL of the extract prepared beforehand (substituted with extraction solvent in the control) was combined with 160 μL of extraction solvent. Additionally, 1 mL of Folin-Ciocâlteu’s reagent water solution (in a ratio of 1:9, v/v) and 800 μL of Na2CO3 solution (prepared by dissolving 1.875 g of Na2CO3 in 25 mL of deionised H2O) were added. The samples were left in the dark for 30 min, and then absorbance at 760 nm was measured with a spectrophotometer. Results were expressed as mg of gallic acid equivalent per 100 g of fresh raspberry (mg GAE/100 g).
FRAP of raspberries was determined by Benzie & Strain [1996] method, subsequently modified by Pellegrini et al. [2003]. A mixture of sodium acetate buffer pH 3.6 (3.1 g of C2H3NaO2 dissolved in 16 mL of CH3COOH and adjusted to 1 L with deionised H2O), 2,4,6-tris(2-pyridyl)-s-triazine (TPTZ) solution (0.0156 g of TPTZ dissolved in 5 mL of 40 mM HCl) and FeCl2 solution (0.135 g dissolved in 25 mL of deionised H2O) was prepared, following a 10:1:1 (v/v/v) ratio. Each sample was then prepared combining 900 μL of the previously prepared FRAP reagent, 90 μL of deionised H2O, 10 μL of raspberry extract (replaced by extraction solvent in the blank) and 20 μL of extraction solvent. The tubes were left in a water bath at 37°C for 30 min to allow the reaction, then absorbance was read at 595 nm. Results were expressed as mmol of ferrous ion per kg of raspberries (mmol Fe2+/kg).
The total anthocyanin content (TAC) determination was based on the method described by Cheng & Breen [1991] with modifications. For each sample, duplicate mixtures were made to measure absorbances at pH of 1 and 4.5. In the first mixture, 50 μL of the extract (replaced with extraction solvent in the control) was combined with 950 μL of pH 1 solution (prepared by dissolving 4.026 g of KCl in 12.45 mL of 12 M HCl and diluted to 1 L with deionised H2O). In the second mixture, 50 μL of the extract (replaced with extraction solvent in the control) was mixed with 950 μL of pH 4.5 solution (prepared by dissolving 32.82 g of sodium acetate in 18 mL of 12 M HCl and diluted to 1 L with deionised H2O). Samples were left in the dark to react for 20 min, then absorbance was read at 515 nm and 700 nm. Results were expressed as mg of cyanidin 3-glucoside (C3G) per 100 g of fruit fresh weight (mg C3G/100 g), according to the following formula from de Souza et al. [2014] with modifications relative to our study:
where: A is the absorbance calculated as A = (A515 − A700)pH1 − (A515−A700)pH 4.5, MW is the molecular weight of C3G (449.384 g/mol), DF is the dilution factor (19), V is the extraction volume, ε is the molar extinction coefficient of C3G (30,400 L/(mol×cm)), l is the optical path length (1 cm), and M is the mass of raspberries used for the extraction.Microbiological analyses
Microbiological analyses were performed only for the raspberries stored at 20°C, comparing controls and UVC-LED treated fruits. A portion of 20 g of randomly selected raspberries were put into a sterile bag with 180 mL of buffered peptone water (Scharlab, Barcelona, Spain) and individually homogenised with a stomacher (Seward, Worthing, United Kingdom) for 30 s, followed by the preparation of serial dilutions with buffered peptone water, up to 10−4. Dilutions between 10−2 and 10−4 were plated (spread plate method) in triplicates on 2 different media, plate count agar (PCA, Scharlab) and yeast glucose chloramphenicol agar (YGC, VWR Chemicals, Milan, Italy), to quantify the total aerobic microbial count and yeasts and moulds, respectively. Plates were incubated at 30°C for 48 h in the incubator (Binder GmbH, Tuttlingen, Germany). Results were expressed as log10 colony forming units (CFU) per g of raspberries.
Statistical analysis
The data from the quality parameters were further processed with a statistical analysis. The two factors considered were the storage temperatures (4°C and 20°C) and the coupled growing system (soil, S; and pot, P) with the treatment (C, control not treated; TR, UVC-irradiated sample). Analysis of variance (ANOVA) was performed, and statistically significant differences were identified by comparison of average values through Tukey’s test (p≤0.05).
RESULTS AND DISCUSSION
Weight loss of raspberries during storage
The WL% were lower for raspberries stored at 4°C than those left at 20°C (Figure 1). Water vapour losses are enhanced by a higher temperature, while refrigeration reduces transpiration [Gimeno et al., 2022; Nunes & Emond, 2007]. The UVC-LED treatment had a significant effect on the weight losses of berries cultivated in soil and then stored at 20°C (Figure 1). The P samples stored under refrigeration showed a similar trend, even if the statistical analysis shows a lack of significant (p>0.05) difference among data. This suggests a possible intolerable stress for the fruits due to the irradiation, independently from the storage temperature, which results in a higher respiration rate, developing greater weight losses [Nguyen et al., 2014]. Moreover, we performed 14-min treatments: this time interval is long from an industrial point of view and also rather stressful for the fruits. The role of the weight losses is particularly important when speaking about fresh fruit, because it is one of the main parameters showing the quality of the product and the economic relevance it may have. Significant weight losses indicate unsuitable storage conditions for berries, leading to wilting and loss of turgidity, which ultimately compromises their visual appeal. Consequently, this results in a less desirable product for consumers.
Figure 1
Percentage weight losses (WL%) of raspberries cultivated in soil (S) and in pots (P) not submitted to UVC-LED treatment (C, control) and irradiated (TR, treated), then stored at 4°C and 20°C for 72 h. Mean values with error bars showing standard deviation are represented. Statistically significant differences are marked with different letters (p≤0.05). Uppercase letters refer to the samples stored at 20°C; lowercase letters refer to the samples stored at 4°C.
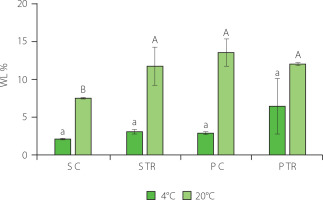
Colour of raspberries
All ∆L*, ∆a*, ∆b* and ∆h° values of the colour analysis of control and UVC-LED treated raspberries after both soil and pot cultivations were above 0 (data not shown), meaning lightness, a*, b* and h° decreased during the storage period of 72 h; however, there were no significant (p>0.05) differences between the samples. This confirms findings earlier described by Gimeno et al. [2022], that is to say the scarce influence of the UV radiation treatment on colour. The visual aspect is, of course, influenced by the colour: a brilliant and vivid tone of the fruit makes the product more attractive for the consumer. Challenges may arise, however, from the deterioration of colour pigments known as anthocyanins, which are sensitive to various storage factors, including temperature [Ochoa et al., 1999]. This sensitivity can result in alterations in colour, predominantly causing a decrease in redness. Commonly, darkening is a typical effect occurring during storage, as a consequence of changes in firmness and weight losses. Tissues undergo modifications and lose water through respiration and transpiration; thus, changes can be observed also in colour [Nunes & Emond, 2007]. Nunes & Emond [2007] found a linear correlation between weight loss and colour; specifically, high weight losses were related to softer and more coloured berries. Furthermore, the stress conditions, such as the UVC irradiation, during the postharvest period can disrupt the physiological degradation processes of berries, causing their accelerated deterioration, even in relation to colour [Nunes & Emond, 2007; Ochoa et al., 1999; Xu & Wu, 2016]. In this case, the treatment was not recognised as visibly altering the colour features of the raspberries.
Texture of raspberries
The indentation in the surface of raspberries and the fact that it is an aggregate of many druplets pose challenges in achieving a uniformly smooth surface for accurate measurements of these fruit texture parameters. Indeed, raspberries show a non-homogeneous structure, with a conical hollow shape, so opposite vectorial forces act on the fruit walls when it is pressed, influencing the measurements. Postharvest textural changes of fruits are related to transpiration. Their extent is different according to the type of fruit and its shape. This discrepancy can commonly be attributed to variations in the composition of the fruit’s outer skin (epicarp) and the surface exposed to the external environment [Rodriguez et al., 2019]. Hardness, chewiness and resilience were the parameters considered for the analysis in our study, because they are more relevant for raspberries [Nunes & Emond, 2007].
Data showed similar hardness of raspberries at start time and after the storage in the climate-controlled room at 20°C, anyway, a statistically significant (p≤0.05) decrease was observed for fruits cultivated in soil and UVC-LED treated (Table 1). Paying attention to the berries stored at 4°C for 72 h, clear reduction (p≤0.05) of hardness was observed compared to the fruits at start time. This can be explained by the fact that senescence results in the softening of tissues. Water losses and, consequently, weight losses are responsible of changes in the fruits’ texture, resulting in a possible wilting of the product. This results in a higher concentration of substances and nutrients in the fruit, changing its structure, causing a possible influence on the consistency of berries [Rodriguez et al., 2019]. For this reason, the results should not be considered as a positive textural improvement. Among the UVC-LED treated raspberries, the soil-cultivated and stored at 20°C samples had the lowest hardness of 1,834 N, which was significantly lower compared to the stored control (2,121 N). Looking at the effect of the UVC-LED treatment on pot-cultivated berries, higher values of hardness were noted for the treated fruits, that was 1,684 N at 4°C and 2,465 N at 20°C compared to 1,585 N and 2,169 N for the controls, respectively; however, the differences were not relevant from a statistical point of view (p>0.05), meaning that it is not possible to associate this effect with the UVC treatment. In the case of soil-cultivated raspberries, no significant (p>0.05) difference was identified, with the exception of a S TR 20 sample, compared to the control ones (S C 20). These mild changes may be due to a possible acceleration of the metabolism of the berries, as also reported by Gimeno et al. [2022] who linked this behaviour to the UV radiation dose and the fruits themselves. A different outcome may be expected as a result of stress, because stressful conditions involve the activation of phenylalanine ammonia lyase (PAL), an enzyme implicated in the synthesis of phenolic compounds and lignin-like polymers, thus resulting in a firmer product. In any case, the great variability and susceptibility of the process with respect to external conditions and intrinsic factors could be the reason why different outcomes were found, showing the necessity of optimisation in relation to the treated matrix [Chawla et al., 2021]. The differences among raspberries and the numerous variables affecting UVC treatment do not always lead to comparable results. What we could suggest is a preventive selection of the fruits according to their maturation stage and dimensions. In fact, the effectiveness of the process relies on many factors both in terms of the treatment and the berries: the species, the cultivar, the maturation stage, the shape and dimensions of the fruit, but also the radiation characteristics, wavelength, distance between the product and the lamps, and the presence of systems moving the fruits [Adhikari et al., 2015; Koutchma, 2014; Singh et al., 2021; Syamaladevi et al., 2015].
Table 1
Texture profile analysis (TPA) results for hardness, chewiness and resilience of raspberries cultivated in soil (S) and in pots (P) at the initial stage (start) and stored at 4°C and 20°C for 72 h after UVC-LED treatment (TR) and without irradiation (C, control).
Chewiness quantifies the product’s resistance to being crushed in the mouth and is linked to elasticity and gumminess. Results of raspberry chewiness measurement are shown in Table 1. Fruits stored at 4°C had lower chewiness compared to the berries at the initial stage. Moreover, at 4°C storage, the UVC-LED treatment was responsible for the better preservation of fruit chewiness, showing a slight but significant (p≤0.05) increment compared to the control samples: 31.5 for the soil-cultivated raspberries (26.8 for the control) and 35.5 for those grown in pots (34.1 for the control). A different situation was definable for the raspberries stored at 20°C, where a less-organised pattern was observed when looking at the results. Insignificant (p>0.05) differences were found between the start values and these determined for the stored samples. In this instance, the irradiated raspberries grown in soil exhibited significantly lower chewiness (34.0) compared to the control fruit (45.2). However, for the pot-cultivated raspberries, chewiness of the irradiated and control berries was statistically comparable (p>0.05). Thus, a correlation can be seen between hardness and chewiness (Table 1). During the UVC-LED treatment, its duration and intensity, together with the characteristics of the fruits themselves, such as the ripening stage, could have acted as enhancers of the senescence process [Gimeno et al., 2022].
The last parameter considered in the TPA analysis was resilience, that is to say the capability of the product to gain its shape back after compression. The UVC-LED treatment did not affect this characteristic (Table 1). Results were also not significantly (p>0.05) different both at the beginning and after storage. This highlights the absence of significant effects associated with the application of UVC light.
Total phenolic content, ferric reducing antioxidant power and total anthocyanin content of raspberries
Regarding the total phenolic content at the start time, the soil-grown raspberries showed a significantly (p≤0.05) higher TPC compared to those cultivated in pots (S start – 197 mg GAE/100 g, P start –131 mg GAE/100 g) (Table 2). This could be attributed to differences in the the composition of the growing substrate: the fact that the pot is a circumscribed space with respect to the field could subsequently result in a different composition in terms of plant bioactive compounds, because water and nutrients are directly in contact with the roots. During refrigerated storage at 4°C, a minor decline in TPC was observed in the soil-cultivated berries compared to the initial measurement (S start). At 4°C storage, the UVC-LED-treated soil-cultivated fruits showed a slightly higher total phenolic content than the control group, but the difference was not statistically significant (p>0.05). In contrast, the plants cultivated in pots exhibited an increase in the TPC during storage, although the final outcome was higher for the control group compared to the irradiated sample. This result may not be considered significant due to the storage temperature, which might hinder the activation and complete development of metabolic pathways involved in phenolic synthesis. Conversely, when stored at 20°C, total phenolic content of raspberries from both soil and pot cultivation exhibited increasing trend after undergoing UVC-LED treatment. Considering the raspberries grown in soil, the total phenolic content increased from 187 mg GAE/100 g for the control to 204 mg GAE/100 g for the radiated berries, although this change was not statistically significant (p>0.05). The fruits grown in pots showed a significant (p≤0.05) increase in the TPC due to the UVC-LED treatment from 158 mg GAE/100 g determined for the control to 200 mg GAE/100 g for the treated berries. These results may be linked to the effect of temperature on the activation of the secondary metabolism of the fruits.
Table 2
Total phenolic content (TPC), ferric reducing antioxidant power (FRAP) and total anthocyanin content (TAC) of raspberries cultivated in soil (S) and in pots (P) at the initial stage (start) and sored at 4°C and 20°C after UVC-LED treatment (TR) and without irradiation (C, control).
[i] Data are reported as mean ± standard deviation. Statistically significant differences are marked with different letters (p≤0.05). Data from soil-grown and pot-grown raspberries was not separated for the statistical analysis. Lowercase letters refer to samples stored at 4°C; uppercase letters refer to samples stored at 20°C. GAE, gallic acid equivalent; C3G, cyanidin 3-glucoside equivalent.
Regarding the FRAP in our research, the raspberries cultivated in soil did not show any noteworthy changes due to irradiation (Table 2). In fact, at both storage temperatures tested, a similar (p>0.05) FRAP was noted for both control and treated samples. In this instance, we cannot observe a discernible effect associated with the exposure of the berries to UVC-LED treatment. Furthermore, the FRAP of berries at the start time was insignificantly (p>0.05) higher for the soil-cultivated samples. However, concerning the fruits grown in pots and then UVC-LED-treated, there was a statistically significant (p≤0.05) increase in FRAP of the treated samples compared to the control group at 20°C. This increase correlated with the rise in total phenolic content, indicating that phenolics were responsible for FRAP of raspberries. The refrigerated storage (4°C) of the treated sample led to a final value of 76.2 mmol Fe2+/kg compared to the 69.9 mmol Fe2+/kg reached by the control. The higher storage temperature, 20°C, enhanced the metabolisms, already promoted by the lighting treatment, and led to FRAP of 78.6 mmol Fe2+/kg, while the control showed 55.3 mmol Fe2+/kg. Increased FRAP may be due to the enhancement of the secondary metabolic pathways involved in antioxidant production because of the UVC-LED treatment, which acts as a stressful event on the fruits [Li et al., 2019]. The initial measurement of FRAP revealed higher values for raspberries cultivated in the soil than in pots, which was consistent with the findings observed in the TPC quantification.
In line with the TPC and FRAP, the TAC was observed to be higher in S start compared to P start (Table 2). This difference was statistically significant (p≤0.05). The TAC differences at 4°C and 20°C for both soil-grown and pot-grown raspberries reflected what was already discussed for TPC and FRAP. Storage at refrigeration temperature (4°C) was not suitable for the development and improvement of the total anthocyanin content with respect to time start. Moreover, the TAC of the control samples was insignificantly (p>0.05) higher than the UVC irradiated berries (S C 4 – 28.9 mg C3G/100 g and S TR 4 – 23.7 mg C3G/100 g; P C 4 – 30.0 mg C3G/100 g and P TR 4 – 25.5 mg C3G/100 g). No differences can be distinguished between the two growing methods. In the case of fruit storage at 20°C, the UVC-LED treatment resulted in a not statistically significant (p>0.05) increase of the TAC compared to control soil-grown raspberries. TAC differences between the berries in starting point and after the storage at 20°C were: S C 20 – 39.0 mg C3G/100 g and S TR 20 – 41.9 mg C3G/100 g out of the starting value of 24.0 mg C3G/100 g; P C 20 – 32.3 mg C3G/100 g and P TR 20 – 34.6 mg C3G/100 g out of the starting value of 18.4 mg C3G/100 g. UV light treatment in postharvest has been recognised as a bioactive compound-enhancing technique, able to increase the level of the main phytochemicals in the berries [Gimeno et al., 2022].
The results presented and discussed in this paragraph can be only partially compared to other data from literature because the UVC-LED treatment has not been performed widely on raspberries yet. Gimeno et al. [2022] treated raspberries with two different UVC doses (2 kJ/m2 and 4 kJ/m2) and then stored them at 6°C for 4, 8 and 12 days. Considering the TPC, their results are comparable to ours, since they observed a modest growth after 4 days of storage. Longer storage period, on the contrary, affects negatively the total phenolic content according to the aforementioned authors [Gimeno et al., 2022]. The FRAP assay highlighted a reduction in the antioxidant activity of raspberries according to Gimeno et al. [2022], which is partially in agreement with the results obtained in our study, where only pot-cultivated samples showed increased FRAP after the UVC radiation treatment compared to the control fruits. UVC radiation treatment has also been applied on other matrices, for example strawberries [Li et al., 2019] and blueberries [Perkins-Veazie et al., 2008]. Fresh-cut strawberries showed a significant increase in bioactive compound content when treated with a UVC dose of 4 kJ/m2 and subsequently stored at 4°C for 7 days. Total phenolic content, total anthocyanin content and antioxidant activity, monitored through the DPPH assay, were positively affected by the light treatment [Li et al., 2019]. Cultivar-specific behaviour was demonstrated in a study by Perkins-Veazie et al. [2008], where Bluecrop blueberries and Collins blueberries showed a different response to the same UVC treatments of 1, 2 and 4 kJ/m2 followed by a storage at 5°C for 7 days and at 20°C for 2 days. Only Bluecrop blueberries had higher TPC and TAC, conversely Collins blueberries were negatively affected by UVC rays, thus their TPC and TAC decreased. After the UVC treatment, TAC not only grew for fresh-cut strawberries [Li et al., 2019] and for Bluecrop blueberries [Perkins-Veazie et al., 2008], but also for raspberries, especially after 4 and 8 days of storage [Gimeno et al., 2022]. This behaviour acknowledged in the literature data and shared by different fruit matrices is opposite to what we measured in our experiment, that is to say the absence of differences after the 3-day storage at 20°C and a lower total anthocyanin content after the storage at 4°C with respect to control groups. From these data we could suppose a possible impact of the radiation dose, which is particularly high in our study (11.90 kJ/m2) with respect to the doses of 1, 2 and 4 kJ/m2 used in the other researches [Gimeno et al., 2022; Li et al., 2019; Perkins-Veazie et al., 2008].
Microbial count of raspberries
We decided to perform microbial analyses only for the raspberry groups stored at 20°C because the majority of the microorganisms representing the fruit microbiota would not grow consistently at 4°C. A positive decontamination effect due to the UVC light exposure of raspberries was not found. No statistically different (p>0.05) results were recorded for C and TR samples (Figure 2). Results from Butot et al. [2018] study demonstrated that the UVC treatment of berries was not able to reduce the load of the inoculated pathogens by more than 1 log10 CFU/g. Moreover, additional time of exposure did not cause a further reduction in the contamination, probably because of the structure and shape of the berries, with cavities protecting the microorganisms. Another result consistent with our findings was reported by Gimeno et al. [2022], who demonstrated the necessity of coupling the UVC treatment and modified atmosphere packaging to delay the fungal growth and to extend the raspberry shelf-life.
Figure 2
Total aerobic microbial, yeast and mould counts of raspberries cultivated in soil (S) and in pots (P), not submitted to UVC-LED treatment (C, control) and irradiated (TR, treated), then stored at 20°C. Mean values with error bars showing standard deviation are represented. Statistically significant differences are marked with different letters (p≤0.05). Lowercase letters refer to the total aerobic microbial count; uppercase letters refer to the yeast count; Greek letters refer to the mould count.
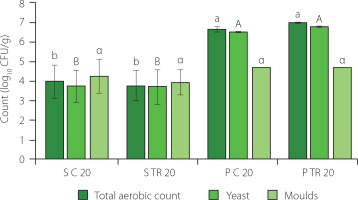
When considering the results from microbiological analysis, it becomes evident that the raspberries cultivated in pots exhibited a higher (p≤0.05) contamination (Figure 2). In the case of the soil-grown berries, both the control and treated fruits exhibited a total aerobic microbial count of 3.98 and 3.77 log10 CFU/g, respectively. These values were approximately 3 log10 lower than the contamination levels observed in the pot-grown berries, which recorded 6.64 log10 CFU/g for the control group and 6.95 log10 CFU/g for the UVC-LED treated group. Similar results were collected for the yeast counts, where the control and irradiated samples from soil cultivation showed a contamination of 3.73 and 3.70 log10 CFU/g, respectively, while the yeast count determined for raspberries grown in pots was 6.50 and 6.73 log10 CFU/g, respectively. The pot-grown raspberries showed a higher microbial load than the soil-grown ones also in this case.
The disparity in microbial counts between the two types (S and P) of raspberries is likely attributable to differences in the preharvest management practices used in the cultivations, leading to varying degrees of contamination. A possible problem could be the watering management and the quality of the water used; all the other procedures, manipulation, and transfers followed the same protocols and were performed in the same way for both soil- and pot-cultivated berries. Another possible contamination problem could derive from the potting soil employed. In addition to this, the pot management system could lead to water stagnation, creating the best conditions for the multiplication of bacteria and fungi. In any case, did the results show microbial counts were higher than 3.5 log10 CFU/g, which indicates some critical issues in primary production. This is because literature references present a total bacteria population of 2.5 log10 CFU/g as the normal microbiota for fresh raspberries [Xu & Wu, 2016]. In fact, the aggregate of drupelets composing the small fruit can create shielded places where microorganisms can grow.
Regarding the mould contamination, no significant difference was detected among the various samples (Figure 2). Their count was similar in all the raspberries, from both soil and pot cultivation, and the UVC-LED treatment was unable to reduce the mould populations compared to control groups.
CONCLUSIONS
According to weight loss results, the UVC-LED treatment of raspberries for 14 min may be stressful for the fruits. The colour of raspberries was not influenced by UVC radiation, and minimal textural changes were found; hence, a direct link could not be clearly stated between these quality parameters of berries and the UVC light treatment. The outcomes obtained were influenced by the storage temperatures. The treatment with UVC-LED rays influenced the metabolic activity of raspberries, determining positive effects, such as the increment of total phenolic content of raspberries cultivated in soil, UVC-LED treated and stored at 4°C, and for those irradiated and stored at 20°C and the increment of ferric reducing antioxidant power for the pot-grown raspberries. The increased level of anthocyanins was not significant. From the microbiological point of view, no antimicrobial effect was detected due to UVC exposure. The microbial population of pot-grown raspberries was notably higher than the one of the soil-grown ones. Conducting additional experimental activities to evaluate the potential impact of irradiation and to define the best approach to be used for every fruit type could be worthwhile, particularly by dividing the storage period into two stages: an initial adaptation phase at 20°C followed by an extended storage period at 4°C. This approach would allow for the activation of secondary metabolism in the fruit while ensuring effective preservation through refrigeration. Considering the supply-chain perspective, the introduction of this technique could create discontinuities with the cold chain approach. It is, therefore, necessary to demonstrate if a possible longer storability and quality maintenance justifies the set-up of a new and partly different management program, comprehensive of the endorsing costs.