INTRODUCTION
Citrus reticulata Blanco is one of the four most cultivated fruits over the world and globally consumed with great popularity [Juric et al., 2023]. ‘Shatangju’ mandarin (Citrus reticulata Blanco cv. Shatangju) is one of the citrus varieties grown extensively in China, especially in southern Chinese provinces of Guangxi, Guangdong and Fujian [Cao et al., 2020]. Recently, the planting area of ‘Shatangju’ mandarin in China was 400,000 ha [Guo et al., 2022]. ‘Shatangju’ mandarin fruits are characterized by attractive appearance, easy-peeling, sweet juiciness, fragrant aroma and other favorable characteristics, and thus become one of the most eagerly consumed fruits [Li et al., 2016]. However, fresh ‘Shatangju’ mandarin fruits are highly susceptible to mechanical damage and pathogen infection due to the thin and crispy peel, juicy flesh, resulting in a large amount of decay and causing serious economic losses [Majerska et al., 2019]. Therefore, how to delay the deterioration of the fruit quality and flavor during transportation and storage, inhibit the occurrence of various postharvest diseases, and prolong the storage time are urgent problems to be solved. The main storage and preservation techniques for ‘Shatangju’ mandarin in China is the use of chemical preservatives, such as pyrimethanil, prochloraz, and iminoctadine triacetate [Chen et al., 2020]. Long-term use of these chemical preservatives will cause microbial resistance on the surface of fruits, leading to their reduced efficacy. Moreover, excessive use will lead to pesticide residues on the fruit surface, posing a potential threat to consumer health.
The application of edible coatings with the environment-friendly advantages and remarkable preservation effect has been widely researched to extend the shelf life and preserve the quality of fresh fruits [Juric et al., 2023]. Starch, chitosan, cellulose, pectin, and their derivatives are the commonly used compounds for fruit coating preservation [Castro-Cegrí et al., 2023]. Edible coatings act as a barrier against oxygen, carbon dioxide and water vapor, delaying the biochemical and physiological changes during storage, reducing microbial growth, and thus effectively maintaining the quality of fresh fruits [Luciano et al., 2022]. The properties of edible coating are largely affected by the type of structural substrate material. The β-cyclodextrin has an amphiphilic, conical, and cylindrical structure, with a hydrophilic outer part (formed by the hydroxyl groups) and a predominantly lipophilic cavity. This special structural property can increase the emulsification of coating agent, making the coating material evenly spread on the surface of fruits to form a film, thus enabling fruit preservation [Matencio et al., 2020]. Glycerol, as a plasticizer of edible starch film, can increase the toughness of film, improve the fluidity of the film liquid, soften the rigid structure of the film, thereby adjust the mechanical properties of the film. Moreover, glycerol also can be used as a polishing agent in food, which can improve the luster of the fruit surface after coating, and improve the transparency of the film [Ben et al., 2022]. Phytic acid has multiple functional groups that can be esterified, complexed and chelated, and can be cross-linked with hydroxyl groups of starch to form esters. The application of phytic acid in fruit and vegetable postharvest preservation coating agents can fully utilize its antioxidant and chelating effects to prevent spoilage caused by oxidation, and seal the stomata of fruits and vegetable epidermis, effectively inhibit the respiration as well as inhibit fungal infection and reproduction [Jiang et al., 2023]. Sodium alginate is a potential biopolymer film or coating component with special colloidal properties, which can thicken, stabilize, suspend, form films, produce gels and stabilize emulsions [Tong et al., 2023]. Therefore, β-cyclodextrin, glycerol, phytic acid and sodium alginate usually can be used as auxiliary materials for edible coating, but there are few studies that have applied them simultaneously in the same coating material.
Due to the high content of amylopectin, cassava starch is highly viscous, and the formed film has the characteristics of high strength, good flexibility, and good barrier [Zhu, 2015]. The cross-linked cassava starch obtained by cross-linking treatment has better shear resistance, better processing strength and stronger film-forming performance [Marques et al., 2006; Wongsagonsup et al., 2014]. However, there are few researches of cross-linked cassava starch-based edible coating on fruits and vegetable preservation, and even fewer researches on the application of cross-linked cassava starch coating in the postharvest preservation of ‘Shatangju’ mandarin, the application technology of which needs in-depth research.
The purpose of this research was, in the first place, to optimize the formulation of a coating agent containing cross-linked cassava starch, β-cyclodextrin, glycerol, and phytic acid by response surface methodology with the comprehensive index of oil permeability (OP) and water vapor permeability (WVP) of coating as responses, and subsequently to investigate the preservation effect of the cross-linked cassava starch-based coating on ‘Shatangju’ mandarins, which would provide a useful technical basis for postharvest preservation of these fruits.
MATERIALS AND METHODS
Cassava starch cross-linking
Cross-linked cassava starch was prepared according to the method described by Wongsagonsup et al. [2014] with some modifications. Native cassava starch purchased from a local starch manufacturer in Hezhou City in China (100 g) was dispersed in 150 mL of a sodium trimetaphosphate solution with different concentrations (0.1%, 0.2%, 0.3%, 0.4% and 0.5%, based on dry weight of starch) so as to obtain a solid content of 40% (w/w). The pH of the starch suspensions was adjusted to 9 with 1 M NaOH solution. The starch suspensions were then incubated at 45°C for 3 h with 120 rpm shaking in a thermostatic oscillating water bath and neutralized to pH 7 with 2 M HCl solution. The modified starches were recovered through centrifugation at 3,200×g for 15 min, washed five times with distilled water and dried at 45°C for 12 h. After that, the dried cross-linked cassava starch samples were pulverized with a universal pulverizer (FW100, Tianjin City Taisite Instrument Co., Ltd., Tianjin, China) and sieved through an 80-mesh sifter. All cross-linked cassava starch samples were kept in airtight polyethylene bags for further analysis of their properties including film thickness, film transmittance, oil permeability and water vapor permeability, which were determined according the procedures described below in the subsection “Determination of properties of cross-linked cassava starch-based films”. Moreover, the sedimentation volume of cross-linked cassava starch samples was determined according to the methods of Chen et al. [2017]. The cross-linked cassava starch with the best properties was selected for further experiments.
Preparation of cross-linked starch coating solution
A portion of 30 g of cross-linked cassava starch with a sedimentation volume of 5.05 mL was dispersed in 970 g of distilled water to obtain a 3 g/100 g starch suspension, then the suspension was incubated at 90°C in a thermostatic oscillating water bath with stirring (400 rpm) to completely gelatinize the starch. The single-factor preparation of cross-linked starch coating solution was performed under the following conditions: the addition levels of β-cyclodextrin of 0.4–1.6 g/100 g, the addition levels of phytic acid of 0.3–1.2 g/100 g, the addition levels of glycerol of 0.3–1.2 g/100 g, and the addition levels of sodium alginate of 0.02–1.00 g/100 g; all the addition levels were based on the dry weight of cross-linked cassava starch. The details of single-factor experiment design are shown in Table 1. After each factor was completed, the optimal level of the factor would be adopted as the test parameter in the following experiment. All materials were stirred (150 rpm) evenly and held at 90°C for 10 min. Subsequently, all materials were stirred by a magnetic agitator (JJ-1, Skyray Instrument Co., Ltd., Changzhou, China) for 20 min with the speed of 500 rpm and then dispersed by a high-speed organization homogenizer (B-500A, Shanghai Yiheng Scientific Instrument Co., Ltd., Shanghai, China) for 2 min with the speed of 15,000 rpm to obtain the coating dispersions, which were then cooled to room temperature and kept in airtight Duran laboratory bottles for further experiments.
Table 1
Design of single-factor experiments for the formulation of cross-linked cassava starch-based coating.
Experimental design
The response surface methodology (RSM) was applied to optimize the cross-linked cassava-based coating agent formulation with the comprehensive index (CI) of OP and WVP of cross-linked cassava starch-based coating as the indicator. CI was calculated according to Equation (1):
Both parameters of OP and WVP were determined as described below.According to the results of single factor test, Box-Behnken design (BBD) was used to determine the optimal addition of β-cyclodextrin (g/100 g, A), phytic acid (g/100 g, B) and glycerol (g/100 g, C) with fixed dosage of 3 g/100 g cross-linked cassava starch. The coded and decoded values of the three independent variables and their levels were shown in Table 2.
Determination of properties of cross-linked cassava starch-based films
The prepared cross-linked cassava starch-based coating dispersions were poured on a horizontally organic glass board and casted evenly. The surface of the organic glass board was required to be clean, dry, and flat. When the surface of the cross-linked cassava starch-based coating film was dried, the organic glass board was placed horizontally in a hot-air oven (DH411C, Yamato, Tokyo, Japan) and left therein at 45°C for 6 h. Afterwards, the film was removed and kept in a desiccator for the future analysis.
Measurement of film thickness
The cross-linked cassava starch-based film was cut into 50×50 mm pieces, and the film thickness was measured at 5 randomly-selected different positions by a spiral micrometer [Yao et al., 2022]. The average value of the measured results was taken as the thickness value of the film.
Measurement of film transmittance
The cross-linked cassava starch-based film was cut into a strip of 10×50 mm and attached to one side of a cuvette. The film transmittance was measured by a visible spectrophotometer (722N, Shanghai INESA Scientific Instruments Co., Ltd., Shanghai, China) at 500 nm with an empty cuvette as the control [Dai et al., 2020].
Determination of oil permeability
Oil permeability (OP) of cross-linked cassava starch-based film was determined according to the method described by Ma et al. [2017] with slight modifications. Briefly, a sheet of uniform and defect-free cross-linked cassava starch-based coating film was cut into a 40×40 mm square and used to seal the mouth of the tube (the diameter was 20 mm) containing 5 mL of soybean oil. The sealed tube was inverted on the weighed medium-speed quantitative filter paper and then placed in a desiccator for 2 days. The filter paper was then weighed and the changes in the weight of the filter paper were recorded. The OP (g×mm/ /m2×d) of cross-linked cassava starch-based coating film was calculated according to Equation (2):
where: ΔW is the change in weight of filter paper (g), d is the thickness of the film (mm), S is the effective area of the film (m2), and T is the number of storage days.Determination of water vapor permeability
Water vapor permeability (WVP) of cross-linked cassava starch-based film was determined according to the method used by Dai et al. [2020] with slight modifications. Briefly, anhydrous calcium chloride was dried at 200°C in a hot-air oven (DH411C, Yamato, Tokyo, Japan) to constant weight. After cooling, 5 g of the dried anhydrous calcium chloride was weighed to a 50-mL conical flask (the diameter was 30 mm), the mouth of conical flask was sealed with a uniform and defect-free cross-linked cassava starch-based coating film, then the total weight of the sealed conical flask was recorded. Finally, the sealed conical flask was placed in a desiccator with a saturated NaCl solution at the bottom to maintain a relative humidity of 75% at 25°C. Because the saturation pressure of water vapor at 25°C is 3.169 kPa and the difference in relative humidity on the both sides of the film was 75%, therefore the vapor pressure difference between the both sides of film was 2.377 kPa. The sealed conical flask was weighed every 12 h until the weight was constant. The WVP (g×mm/m2×d×kPa) of the film was calculated using Equations (3) and (4):
where: ΔP is the vapor pressure difference between the both sides of film (kPa), p is the saturation pressure of water vapor at 25°C (kPa), ΔRH is the difference in relative humidity (%) on both sides of the film, Δm is the change in weight of sealed conical flask (g), d is the thickness of the film (mm), S is the effective area of the film (m2), and T is the number of storage days.Coating treatment of ‘Shatangju’ mandarin
‘Shatangju’ mandarins were harvested at the day of experiment in their natural maturation age from a local orchard in Hezhou City, China, and transferred to the laboratory within 2 h. ‘Shatangju’ mandarins with uniform shape, color and size without bruising or disease symptoms were selected and washed with running tap water and dried at room temperature. All ‘Shatangju’ mandarins were then randomly divided into two groups with 120 fruits each, among which the group without coating treatment was used as the control group. The experimental ‘Shatangju’ mandarins were soaked in cross-linked cassava starch-based coating dispersions for 15 s, and then taken out and dried with electric fan. Afterwards, the coating treatment was repeated three times, and the coated samples were stored at room temperature for 20 days.
Determination of quality indicators of ‘Shatangju’ mandarins during storage period
During the storage period, ‘Shatangju’ mandarins from each group were randomly selected every 2 days for the determination of quality indicators.
Fruit firmness determination
The ‘Shatangju’ mandarins were subjected to texture profile analysis using a texture analyzer (TA.XT PLUS, Stable Micro Systems, London, UK) with a P/2 probe used to measure fruit firmness. The pre-test speed and the test speed were set at 1.0 mm/s, the post-test speed was set at 2.0 mm/s, while the penetration depth was 10 mm. Measurements regarding firmness were taken on the equatorial zone on each fruit at 90°.
Assessment of decay rate
Fruits with black spots, brown spots, mold or at least one leakage of juice on the fruit peel were identified as decayed. The decay rate was calculated as the ratio of the decayed fruit number to the total fruit number [Yang et al., 2019].
Weight loss rate determination
The weight of ‘Shatangju’ mandarins was recorded every 2 days during the storage period. The difference between the initial weight and the weight on the inspection date of the fruit was considered as weight loss, and the results were expressed as percentage [Méndez et al., 2022].
Determination of total soluble solid content
Total soluble solid content of ‘Shatangju’ mandarins was determined with a digital hand refractometer (PR101-a, Atago Co., Ltd., Tokyo, Japan) following the manufacturers’ protocols, and the results were expressed as g per 100 g of fruit.
Titratable acidity determination
The titratable acidity of ‘Shatangju’ mandarins was determined using titration method. In brief, 10 g of ‘Shatangju’ mandarin flesh was grinded in a mortar with 30 mL of distilled water for 5 min, and then the mixture was filtered using qualitative filter paper with 2.5 µm pore size. The filtrate was collected into a volumetric flask and the volume was fixed to 100 mL. Aliquot of 10 mL of the fixed volume filtrate was titrated with 0.1 M NaOH as titrant and phenolphthalein as color indicator. The results were expressed as g of citric acid equivalent per 100 g of fruit [Chettri et al., 2023].
Determination of ascorbic acid content
Ascorbic acid content of ‘Shatangju’ mandarins was determined using 2,6-dichlorophenol-indophenol titrimetric method as previously described [Chettri et al., 2023; Wang et al., 2016a]. Briefly, 10 g of ‘Shatangju’ mandarin flesh was grinded in a mortar with 30 mL of 2% oxalic acid solution for 5 min, and then homogenized in a high-speed organization homogenizer (B-500A, Shanghai Yiheng Scientific Instrument Co., Ltd.) at 12,000 rpm for 3 min. After centrifugation and filtration, 10 mL of the filtrate were collected and titrated with 2,6-dichlorophenol-indophenol solution until pink color for 15 s, and 10 mL of 2 g/100 g oxalic acid solutions were titrated as blank control titration. The results were calculated based on the standard curve of ascorbic acid and expressed as mg per 100 g of fruit.
Determination of respiration intensity
Static method was used to determine the respiration intensity of ‘Shatangju’ mandarin fruit during the storage period [Saltveit, 2019]. In brief, the culture dish containing 10 mL of 0.4 M NaOH solution was placed at the bottom of the desiccator, next 1,000 g of ‘Shatangju’ mandarin were placed on the partition plate, and then the desiccator was sealed immediately and left static at room temperature (25°C) for 1 h. After standing static for 1 h, the solution in culture dish was transferred into a triangular flask (rinsed with distilled water 4–5 times), the 5 mL of a saturated BaCl2 solution and 2 drops of phenolphthalein were added, the mixture was titrated with 0.2 M oxalic acid, the usage amount of oxalic acid was recorded, and blank control was titrated with the same method. Respiration intensity (RI, mg/kg×h) of ‘Shatangju’ mandarin fruit was calculated according to the following Equation (5):
where: C is the concentration of NaOH solution (0.2 M), V1 is the usage amount of NaOH solution for titration of blank control (mL), V2 is the usage amount of NaOH solution for titration of sample (mL), W is sample mass (g), T is measurement time (1 h), and 22 is mass conversion coefficient of NaOH and CO2 in the determination.Statistical analysis
Data were recorded as mean and standard deviation (n≥3). The analysis of variance (ANOVA) conducted by Duncan’s test was used to examine the differences between samples (p<0.05). All statistical analyses were done using Data Processing System (7.05 for Windows, Hangzhou Ruifeng Info-technology Co., Zhejiang, China) and Microsoft Office Excel 365 (Microsoft Corp., Redmond, WA, USA).
RESULTS AND DISCUSSION
Effect of different cross-linking degree on properties of the cross-linked cassava starch film
It is difficult to measure the cross-linking degree of cross-linked starch, but the cross-linking degree is negatively correlated with sedimentation volume. In other words, the sedimentation volume decreases in the highly cross-linked starches with increasing amounts of the cross-linking reagent; therefore, the sedimentation volume is usually used to indicate the level of cross-linking degree [Kou & Gao, 2018]. Five cross-linked cassava starch samples with different cross-linking degree were prepared according to the method described in the section of Material and Methods. The sedimentation volume of the prepared cross-linked starches was 7.05 mL, 6.50 mL, 5.90 mL, 5.55 mL, and 5.05 mL, respectively. Correspondingly, the five cross-linked cassava starch samples were named as CS7.05, CS6.50, CS5.90, CS5.55, and CS5.05. The thickness of films of these cross-linked cassava starches was between 0.0358 mm and 0.0376 mm, but the OP and WVP decreased with the decrease of sedimentation volume (Table 3). The smaller the sedimentation volume, the better the performance of the cross-linked cassava starch film to prevent water vapor penetration and oil penetration, and the better the film transparency. Moreover, according to the pre-experimental results, the sedimentation volume of the cross-linked cassava starch did not significantly decrease with further increasing the dosage of sodium trimetaphosphate. Therefore, the cross-linked cassava starch (CS5.05) with the sedimentation volume of 5.05 mL was used as the basic raw material of starch-based coating agent in the subsequent experiments.
Table 3
Thickness, transparency, water vapor permeability (WVP) and oil permeability (OP) of films of cross-linked cassava starch with different sedimentation volume.
Effect of β-cyclodextrin on properties the of cross-linked cassava starch-based film
Adding an appropriate amount of β-cyclodextrin to a cross-linked cassava starch-based solution can effectively increase the emulsification effect and make the coating solution to flow more evenly on the surface of the fruits during the coating process to form a more uniform film. The thickness of the cross-linked cassava starch-based film increased with the increasing content of β-cyclodextrin, while the transparency of the film decreased (Table 4). With β-cyclodextrin content increasing from 0.4 g/100 g to 1.6 g/100 g, the OP of the film decreased from 0.173 to 0.128 g×mm/m2×d, and then increased from 0.128 to 0.257 g×mm/m2×d, while the WVP of the film decreased from 2.183 to 2.096 g×mm/m2×d×kPa, and then increased from 2.096 to 2.965 g×mm/m2×d×kPa. At the 1.0 g/100 g addition of β-cyclodextrin, the film had the lowest OP and WVP, which was 0.128 g×mm/m2×d and 2.096 g×mm/m2×d×kPa, respectively, which is correlated with the molecule structural properties of β-cyclodextrin as β-cyclodextrin itself has an average of 16 crystalline water but still can maintain the crystalline complexation [Liu, 2023].
Table 4
Thickness, transparency, water vapor permeability (WVP) and oil permeability (OP) of cross-linked cassava starch-based films with different β-cyclodextrin content.
Effect of glycerol on properties of the cross-linked cassava starch-based film
The thickness of the cross-linked cassava starch-based film fluctuated between 0.0422 and 0.0432 mm with the increasing addition of glycerol, while the transparency of the film decreased (Table 5). With the increasing addition of glycerol from 0.3 g/100 g to 1.2 g/100 g, the OP of the film decreased from 0.210 to 0.164 g×mm/m2×d, and then increased from 0.164 to 0.252 g×mm/m2×d, while the WVP of the film decreased from 2.319 to 2.024 g×mm/m2×d×kPa, and then increased from 2.024 to 2.418 g×mm/m2×d×kPa. When the addition of glycerol was 0.7 g/100 g, the film had lowest OP and WVP, which was 0.164 g×mm/m2×d and 2.024 g×mm/m2×d×kPa, respectively. These results are consistent with the findings reported for sweet potato starch films plasticized with glycerol [Ballesteros-Mártinez et al., 2020] and cassava starch-based nanocomposite films [Ma et al., 2017]. The cross-linked cassava starch-based coating solution can transform into polymer winding phase with the added glycerol, and a strong interaction occurred between glycerol and cross-linked cassava starch, resulting in dense structure, thus reducing the OP and WVP of the film [Mali et al., 2006].
Table 5
Thickness, transparency, water vapor permeability (WVP) and oil permeability (OP) of cross-linked cassava starch-based films with different glycerol content.
However, because glycerol contains three hydrophilic groups, adding excessive glycerol would increase the number of hydrophilic groups of the film, and the WVP would also increase. Excessive glycerol would destroy the starch structure, resulting in the increase of OP and WVP of the film [Ballesteros-Mártinez et al., 2020]. Therefore, in our study, in order to obtain smaller OP and WVP of the film, the suitable addition of glycerol was 0.7 g/100 g.
Effect of phytic acid on properties of the cross-linked cassava starch-based film
Phytic acid can chelate with polyvalent metal ions in food coenzyme, inhibit or delay the enzymatic reaction, thus ensuring the purpose of fruit preservation [Feizollahi et al., 2021]. The thickness of the cross-linked cassava starch-based film fluctuated between 0.0424 and 0.0432 mm with the increasing addition of phytic acid, while the transparency of the film decreased (Table 6). With phytic acid content increasing from 0.3 g/100 g to 1.2 g/100 g, the OP of the film decreased from 0.174 to 0.139 g×mm/m2×d, and then increased from 0.139 to 0.165 g×mm/m2×d, while the WVP of the film decreased from 2.203 to 2.079 g×mm/m2×d×kPa, and then increased from 2.079 to 2.283 g×mm/m2×d×kPa. When the addition of phytic acid was 0.7 g/100 g, the film had lowest OP and WVP, which was 0.139 g×mm/m2×d and 2.079 g×mm/m2×d×kPa, respectively. Therefore, in order to obtain lower OP and WVP of the film, the suitable addition of phytic acid was 0.7 g/100 g.
Table 6
Thickness, transparency, water vapor permeability (WVP) and oil permeability (OP) of cross-linked cassava starch-based films with different phytic acid content.
Effect of sodium alginate on properties of the cross-linked cassava starch-based film
The thickness of the cross-linked cassava starch-based film increased from 0.0426 to 0.0446 mm with the increasing addition of sodium alginate, while the transparency of the film generally decreased from 85.67 to 81.40% (Table 7). The OP of the film fluctuated between 0.151 and 0.154 g×mm/m2×d, while WVP slightly decreased first and then increased with the increasing addition of sodium alginate. When the addition of sodium alginate was 0.06 g/100 g, the film had lowest WVP (2.069 g×mm/ m2×d×kPa) and there were no significant differences (p≥0.05) between the samples in terms of OP values. Therefore, in order to obtain smaller WVP of the film, the suitable addition of sodium alginate was 0.06 g/100 g. Since sodium alginate has the ability to enhance the viscosity of solution and causes the gel property in the medium [Aggarwal et al., 2020; Khoshdouni Farahani et al., 2023], its small amount added would make the viscosity of the cross-linked cassava starch-based coating liquid sharply increased, resulting in difficulty of coating liquid to cast evenly and form well. Therefore, sodium alginate was not selected as an auxiliary agent of the cross-linked cassava starch-based coating in the subsequent research.
Table 7
Thickness, transparency, water vapor permeability (WVP) and oil permeability (OP) of cross-linked cassava starch-based films with different sodium alginate content.
Optimization of the cross-linked cassava starch coating formulation by response surface methodology
According to the analysis of the single-factor experiment, the three-factor and three-level test was proceeded by RSM. Design of the experiment and the results of the dependent variable for the formulation of the cross-linked cassava starch coating are shown in Table 8. The comprehensive index of OP and the WVP of the cross-linked cassava starch-based coating was influenced by the addition of β-cyclodextrin, phytic acid and glycerol. A second-order regression equation was established to fit with the experimental data as the following Equation (6):
where: Y is comprehensive index of OP and WVP; and A, B, C are the addition levels of β-cyclodextrin, phytic acid and glycerol, respectively.Table 8
Design of the experiment and the results of the dependent variable for the formulation of cross-linked cassava starch-based coating.
The data were analyzed by ANOVA to assess the significance of those factors and their interactions in this model (Table 9). The credible predictive value (R2=0.9839) showed a high correlation between the predicted value and the experimental value. The adjusted coefficient of the determination was R2Adj=0.9632. The difference between R2 and R2Adj was <0.2, suggesting reasonable agreement between them. The absence of fit term (p=0.0773>0.05) was not significant and the coefficient of variation (C.V.=4.54%) was <5%, indicating that the data had fine fit and reproducibility. In addition, the coefficients of AB, BC, A2, B2 and C2 were significant, with a low p-value (p<0.05), but the coefficient of AC was insignificant with a high p-value (p>0.05).
Table 9
Variance analysis of response surface test.
Source of variation | Sum of squares | Degree of freedom | Mean square | F-value | p-value |
---|---|---|---|---|---|
Model | 1.49 | 9 | 0.17 | 47.57 | <0.0001** |
A | 0.023 | 1 | 0.023 | 6.59 | 0.0371* |
B | 0.011 | 1 | 0.011 | 3.2 | 0.1166 |
C | 0.12 | 1 | 0.12 | 34.39 | 0.0006** |
AB | 0.039 | 1 | 0.039 | 11.17 | 0.0124* |
AC | 0.00613 | 1 | 0.00613 | 1.76 | 0.2263 |
BC | 0.052 | 1 | 0.052 | 15.04 | 0.0061** |
A2 | 0.67 | 1 | 0.67 | 192.78 | <0.0001** |
B2 | 0.22 | 1 | 0.22 | 62.33 | <0.0001** |
C2 | 0.23 | 1 | 0.23 | 66.8 | <0.0001** |
Residual | 0.024 | 7 | 0.00348 | ||
Lack of fit | 0.019 | 3 | 0.00641 | 4.99 | 0.0773 |
Pure error | 0.00514 | 4 | 4 | ||
Correlation total | 1.52 | 16 | |||
R2 | 0.9839 | ||||
R2Adj | 0.9632 |
The interaction of the three independent variables of the addition levels of β-cyclodextrin, phytic acid and glycerol was used to plot the response surface curves and the contour line for the comprehensive index of OP and WVP, as shown in Figure 1. The steepness of the response surface curves reflects the effects between the variables on the response value, and the shape of the corresponding contour plot indicates whether the interactions between the independent parameters are significant [Cai et al., 2019; Wang et al., 2016b]. When the response surface curves have greater inclination, the slope is steeper, it means the variables have greater effects on the response value. An oval contour plot indicates a significant interaction between the two variables, while a circular contour plot indicates an insignificant interaction between the two variables. The response surface plot for β-cyclodextrin and phytic acid as variables was steep, and the contour plot was oval, indicating that the comprehensive index of OP and WVP was sensitive to the changes of the two variables and that the interaction was significant (Figure 1A and B). These plots were similar to those for glycerol and phytic acid as variables (Figure 1E and F). In turn, the response surface plot of β-cyclodextrin and glycerol was relatively steep (Figure 1C), indicating that the comprehensive index of OP and WVP was sensitive to the changes of the two variables. The contour plot for these variables was circular (Figure 1D), suggesting that the interaction was insignificant. Based on the above analyses, the addition of β-cyclodextrin, phytic acid and glycerol was found to be highly important for the OP and WVP of the cross-linked cassava starch-based coating.
Figure 1
Response surface plots (A, C, E) and contour plots (B, D, E) of the interactions between β-cyclodextrin, phytic acid and glycerol used in the cross-linked cassava starch-based formulation. OP, oil permeability; WVP, water vapor permeability.
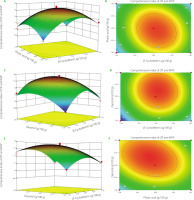
The analysis of the response surface method results provided the following optimal conditions using the model equation: the addition of β-cyclodextrin, phytic acid and glycerol was 0.7%, 0.5% and 0.5%, respectively. Under optimal conditions, the minimum predicted comprehensive index of OP and WVP of the cross-linked cassava starch-based coating was 0.455. To verify the predicted result, a verification experiment was carried out under the optimal conditions with a fixed dosage of 3 g/100 g of the cross-linked cassava starch. As a result, actual OP of 0.0883±0.0001 g×mm/m2×d and actual WVP of 0.8196±0.0004 g×mm/m2×d×kPa were obtained, meaning that the actual comprehensive index of OP and WVP was 0.454±0.0002. The error between the actual comprehensive index of OP and WVP (0.454±0.0002) and predicted value (0.455) was 0.22%, indicating that the formulation of the cross-linked cassava starch-based coating agent optimized by RSM had good repeatability and that the model designed in this study was suitable for predicting the comprehensive index of OP and WVP of the cross-linked cassava starch-based coating.
Effect of the cross-linked cassava starch-based coating on the preservation of ‘Shatangju’ mandarins
The optimized formulation of the cross-linked cassava starch-based coating had been applied on the fresh ‘Shatangju’ mandarins to investigate the effects on fruit postharvest preservation, with uncoated ‘Shatangju’ mandarins as the control. The main function of coating was to reduce the gas exchange of fruit and slow down the postharvest physiological processes [Cao et al., 2022]. Firmness is one of the important indicators of the quality of fresh fruit during postharvest storage. As shown in Figure 2A, the firmness of ‘Shatangju’ mandarins in both the experimental and control groups decreased with the extension of storage time, which was consistent with the previous articles reporting that edible coating was effective in maintaining fruit firmness of ‘Dottato’ fig (Ficus carica L.) and Huangguan pears [Allegra et al., 2017; Dai et al., 2020]. The ‘Shatangju’ mandarins in the control group without coating had significantly lower firmness than that of the experimental group with coating since the 2nd day of storage. On the 10th day of storage, the firmness of ‘Shatangju’ mandarins in the control group was 4.55 N, while the firmness of fruits with the coating was 5.07 N (1.11 times that of the control group). On the 20th day of storage, the firmness of ‘Shatangju’ mandarins without coating was 1.75 N, while the firmness of coated ‘Shatangju’ mandarins was 1.93 times higher (3.37 N). These results show that the cross-linked cassava starch-based coating can effectively maintain the firmness of postharvest ‘Shatangju’ mandarins.
Figure 2
Firmness (A), decay rate (B), weight loss rate (C), soluble solid content (D), titratable acidity (E), ascorbic acid content (F) and respiration intensity (G) of ‘Shatangju’ mandarins uncoated (control group) and coated with the cross-linked cassava starch-based coating agent (experimental group) during storage. All values are presented as the mean and standard deviation. Different letters (a and b) within the same storage time indicate significant differences between experimental and control group (p<0.05).
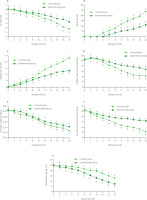
Microorganisms exist widely in nature, and a certain amount of putrefactive microorganisms exist on the surface of fruits after being harvested. In arid and sub-tropical climates, significant postharvest decay in Citrus reticulata was reported to be caused primarily by Penicillium digitatum, and secondarily by P. italicum and Geotrichum citri-aurantii (syn. G. candidum) [Hong et al., 2014]. Therefore, the decay rate directly reflects the appearance quality of ‘Shatangju’ mandarins during the postharvest storage. As shown in Figure 2B, there were no rotten fruits in both the coated experimental group and the uncoated control group on the 4th day of storage, the decay rate of was 0%. With the extension of storage time to the 6th day, ‘Shatangju’ mandarins in the control group had rotten fruits with a decay rate of 6.67%, while the coated ‘Shatangju’ mandarins had rotten fruits on the 8th day of storage with a decay rate of 4.00%. The decay rate of ‘Shatangju’ mandarins of both groups showed an increasing trend with the extension of storage time, but the decay rate of the coated ‘Shatangju’ mandarins was always significantly (p<0.05) lower than that of the uncoated fruits. In the middle and late storage period (10th–20th day), the decay rate of the ‘Shatangju’ mandarins in the control group increased rapidly, and was 48.00% on the 20th day of storage, while that in the coated experimental ‘Shatangju’ mandarins was only 22.67%, which was lower than that in the uncoated fruits on the 12th day of storage (the decay rate was 24.00%). All these results indicate that the cross-linked cassava starch-based coating treatment can effectively reduce the decay rate of ‘Shatangju’ mandarins and prolong its shelf life at room temperature.
During storage, transpiration and respiration of fruits can cause the loss of water and carbon atoms, leading to a rapid loss of internal moisture, resulting in wilting and shriveling of the fruit surface and continuous reduction of fruit weight, which not only affect the taste, but also reduce the commercial value and shorten the shelf life of the fruit [Mukama et al., 2019]. The weight loss rate of ‘Shatangju’ mandarins increased during the storage period, and the weight loss rate of coated ‘Shatangju’ mandarins was significantly (p<0.05) lower than that of the uncoated fruit, especially in the middle and late storage period (10th–20th day) (Figure 2C). The weight loss rate of ‘Shatangju’ mandarins in the coated group was 7.81%, which was 43.40% lower than that in the uncoated control group (13.8%) at the same time. These weight loss rate results were consistent with literature data showing that the fruit weight loss rate increased during storage and the coating treatment was beneficial in the maintenance of fruit weight [Dai et al., 2020]. Coating treatment can form a uniform and transparent protective film on the surface of fruits. This film may act as a physical barrier on the surface of fruits, and can prevent the water loss of fruits, which was consistent with the results indicating that the film can effectively reduce the WVP.
The changes in the content of soluble solids can affect the taste and the quality of fruit to some extent. Soluble solids are regarded as a substrate of postharvest respiration of fruits, while lower respiration intensity is reasonable to maintain a relatively high soluble solids content. The soluble solid content of ‘Shatangju’ mandarins in both the coated and uncoated groups increased at the initial stage of storage, and then subsequently decreased with the extension of storage time (Figure 2D), which was consistent with previous results reported for Shatang mandarin fruits with chitosan/nanocrystal cellulose coatings [Cao et al., 2022]. During the storage, the soluble solid content of ‘Shatangju’ mandarins in the group with the coating was higher than that of the control group without the coating, especially in the middle and late storage, which was consistent with the results indicating that the coated ‘Shatangju’ mandarins had relatively lower respiration intensity. The results might be due to the fact that the coating treatment can form a film that can effectively inhibit respiration and reduce the decrease in soluble solid content of ‘Shatangju’ mandarin caused by respiration. On the 20th day of storage, the soluble solid content in the uncoated control group was 8.23%, while in the coated fruits it was 10.32%, which was 1.32 times of that in the control group.
The titratable acidity affects the fruit taste, which reflects the sensory quality and the commercial value of fruit. ‘Shatangju’ mandarins used organic acids as the substrate for respiration, which led to the decrease of their titratable acidity with the prolongation of the storage time (Figure 2E). The titratable acidity of the coated fruits was significantly (p<0.05) higher than that of the control group after the 14th day of storage. The surface of ‘Shatangju’ mandarins in the experimental group was coated with transparent protective film which isolated it from air, inhibited the respiration and effectively slowed down the consumption of organic acids, thus ensuring the preservation effects.
The titratable acidity affects the fruit taste, which reflects the sensory quality and the commercial value of fruit. ‘Shatangju’ mandarins used organic acids as the substrate for respiration, which led to the decrease of their titratable acidity with the prolongation of the storage time (Figure 2E). The titratable acidity of the coated fruits was significantly (p<0.05) higher than that of the control group after the 14th day of storage. The surface of ‘Shatangju’ mandarins in the experimental group was coated with transparent protective film which isolated it from air, inhibited the respiration and effectively slowed down the consumption of organic acids, thus ensuring the preservation effects.
The titratable acidity affects the fruit taste, which reflects the sensory quality and the commercial value of fruit. ‘Shatangju’ mandarins used organic acids as the substrate for respiration, which led to the decrease of their titratable acidity with the prolongation of the storage time (Figure 2E). The titratable acidity of the coated fruits was significantly (p<0.05) higher than that of the control group after the 14th day of storage. The surface of ‘Shatangju’ mandarins in the experimental group was coated with transparent protective film which isolated it from air, inhibited the respiration and effectively slowed down the consumption of organic acids, thus ensuring the preservation effects.
Vitamin C is extremely unstable, easily soluble in water and easily to be oxidized. It is a very important nutritional component of ‘Shatangju’ mandarins [Chen et al., 2020]. The changes of ascorbic acid content in ‘Shatangju’ mandarins can reflect the nutritional status during the postharvest storage. The content of ascorbic acid of ‘Shatangju’ mandarins in both the coated and uncoated fruits continuously decreased with storage time prolongation, with the decline in the ascorbic acid content during postharvest storage being consistent with results of a previous study on strawberries stored at 20°C [Shin et al., 2007]. However, the content of ascorbic acid of coated ‘Shatangju’ mandarins was significantly (p<0.05) higher than that of the control group (Figure 2F). The ascorbic acid content in the uncoated ‘Shatangju’ mandarins on the 20th day of storage was 8.09 mg/100 g, which was only 50% of the ascorbic acid content of fresh fruits (stored for 0 day). In turn, the ascorbic acid content in the coated ‘Shatangju’ mandarins was 12.27 mg/100 g on the 20th day of storage, which was 75% of its content in fresh ‘Shatangju’ mandarin, and it was 1.52 times of the ascorbic acid content in the control group. These results suggest that the cross-linked cassava starch-based coating could slow down the degradation of ascorbic acid, thus may improve the antioxidant capacity of ‘Shatangju’ mandarins.
The intensity of postharvest respiration of fruits and vegetables is an important characteristic of the rate of metabolism during storage and can be used as an indicator of storage potential, which is directly related to the quality changes, physiological diseases and shelf life of fruits and vegetables [Wantat et al., 2022]. The greater the postharvest respiration intensity of fruits and vegetables, the shorter their shelf life [Khalid et al., 2017]. Figure 2G shows that fresh ‘Shatangju’ mandarins (stored 0 day) had high respiration intensity, and the respiration intensity of both coated and uncoated ‘Shatangju’ mandarins showed a decreasing trend with the prolonged storage, indicating that ‘Shatangju’ mandarin was not a kind of climacteric fruit. The respiration intensity of fruits of the coated experimental group was always lower than that of the control group over the whole storage period, especially at the late stage of storage. The respiration intensity of coated ‘Shatangju’ mandarins was 7.29 mg/kg×h, which was 42.06% lower than that of the control fruits (12.58 mg/kg×h). This result was consistent with findings from other research showing the ability of an edible coating to lower respiration intensity in fruits [Nasrin et al., 2017]. These results indicated that the cross-linked cassava starch-based coating could effectively reduce the respiration intensity and prolong the postharvest life of ‘Shatangju’ mandarins.
CONCLUSIONS
In this study, response surface methodology was successfully applied to optimize the formulation of the cross-linked cassava starch-based coating agent. The optimum conditions for the formulation with a fixed dosage of 3 g/100 g of cross-linked cassava starch were as follows: the addition of β-cyclodextrin was 0.7 g/100 g, the addition of phytic acid was 0.5 g/100 g and the addition of glycerol was 0.5 g/100 g. Optimization and model validation data showed that the experimental results were reliable and the model established within the range of experimental conditions was accurate and effective.
In addition, the results achieved from preservation tests of ‘Shatangju’ mandarin with the cross-linked cassava starch-based coating agent indicated that the cross-linked cassava starch-based coating solution could form a transparent film on the surface of ‘Shatangju’ mandarins after the coating treatment. The transparent film effectively improved the changes in firmness, decay rate, weight loss rate, soluble solid content, titratable acidity and ascorbic acid content during the storage period. The coating treatment effectively delayed the postharvest respiration of ‘Shatangju’ mandarins. All the postharvest experiment results indicate that the cross-linked cassava starch-based coating agent applied on the surface of ‘Shatangju’ mandarins effectively reduced the loss of nutrients, delayed wilting, shriveling and decay of fruit, prolonged the postharvest preservation period, and thus improved their commercial value.