INTRODUCTION
Mango (Mangifera indica L.) is a tropical fruit with high production and consumption in the world due to its attractive sensorial attributes and nutritional value [Maldonado-Celis et al., 2019]. It is a source of dietary fiber, vitamins, carbohydrates, fatty acids, and amino acids as well as presents a considerable biological activity by the high content of phenolic compounds, terpenoids, sterols, and carotenoids [Kumar et al., 2021]. The main producing regions of mango in the world are Asia (60% of world production), Latin America (25%), and Africa (14%) [Santo et al., 2018]. There are more than 1,000 mango cultivars in the world, but the main cultivars harvested in Latin America for commercialization are ‘Kent’, ‘Keitt’, ‘Ataulfo’, ‘Tommy Atkins’, and ‘Haden’ [Kansci et al., 2008]. ‘Tommy Atkins’ cultivar is one of the most appreciated as it is characterized by its large size and color, its resistance to handling, and its potential for minimal processing [Djioua et al., 2010].
Fresh-cut fruits have similar properties to fresh fruits, and their consumption is implied to confer multiple benefits for human health, including the prevention of different chronic, degenerative, and cardiovascular diseases [Artés-Hernández et al., 2017]. However, these products are highly susceptible to microbial growth because their protective tissue is removed and cell contents are released during cutting operation, thereby they serve as a substrate for microorganisms that cause decay and development of human pathogens [Artés-Hernández et al., 2021]. Accordingly, it is important to comply with strict hygiene and manufacturing practices and to recognize critical control points during processing [De Corato, 2020]. For storage, it is necessary to ensure low temperatures and a packaging to control the fresh-cut fruit metabolic activity and accelerated microbial growth. Modified or controlled atmosphere are usually applied using polymeric films and containers, which allows to prevent the flow of respiratory gases, reduce O2 concentration, and rise CO2 concentration in the package headspace [Giannakourou & Tsironi, 2021].
With the increase in the consumption of fresh-cut fruits, a concern has arisen regarding the effectiveness of disinfection methods in reducing microorganisms that cause foodborne diseases [Hinojosa et al., 2013]. Different chemical and physical methods have been studied for the disinfection of fresh-cut fruits. The ultraviolet short wave (UV-C) irradiation is an emerging physical technology that inactivates microorganisms and simultaneously induces defense mechanisms in plant. This leads to the production of phytoalexins after treatment and allows to improve the conservation and attributes of minimally-processed fruits [Zambrano-Zaragoza et al., 2021]. These effects depend on the dose of UV-C, although the beneficial effects are attributed to short doses (low dose of radiation or short exposure times).
Different studies have indicated that UV-C treatment preserves quality attributes of fresh-cut mango during storage. González-Aguilar et al. [2007] found that irradiation of fresh-cut ‘Tommy Atkins’ mango for 1 to 10 min induced the accumulation of phenolics and increased antioxidant capacity during storage for 15 days at 5°C. UV-C treatment of ‘Kent’ mango slices for 30 s had a positive effect on the physicochemical characteristics and produced lower counts of mesophiles, psychrophiles and molds and yeasts, compared to control samples [Márquez-Villacorta et al., 2011]. Irradiation of fresh-cut ‘Kent’ mango with 14 kJ/m2 resulted in lower counts of mesophiles and molds and yeasts, and an increment of contents of total phenolics and flavonoids during 15 days of storage at 5°C [Márquez-Villacorta & Pretell-Vásquez, 2013]. However, the treatment doses should be determined empirically for the specific research material. In our preliminary studies, an optimal UV-C dose of 6 kJ/m2 was estimated for the inactivation of less than 2 log cfu/g of Escherichia coli O157:H7, Salmonella Typhimurium and Listeria monocytogenes on fresh-cut ‘Tommy Atkins’ mango. The aim of this research was to evaluate the effects of this UV-C dose on the safety and quality attributes of fresh-cut ‘Tommy Atkins’ mango during refrigerated storage.
MATERIALS AND METHODS
Plant material
Mango fruits (Mangifera indica L. cv. ‘Tommy Atkins’) in stage 3 of maturity were purchased from a Hermosillo (México) local market. Stage of maturity was determined according to the total soluble solid content for this cultivar [Lopes et al., 2021]. Ten kg of fruit with uniform size and maturity were stored at 5°C for 24 h. Subsequently, mangoes were washed, cleaned for 5 min with a 200 mg/L sodium hypochlorite solution, and left to dry in the air.
Fresh-cut mango processing
Peel and seed were removed from fruits. Pulp was cut into 10×60×20 mm spears and divided to three lots. The first lot (C, control) consisted of fresh-cut mango without treatment. The second lot (H) was immersed in a 10 mg/L sodium hypochlorite (NaClO) solution for 1 min and drained for 2 min [Djioua et al., 2010]. A UV-C dose of 6 kJ/m2 established by Rodríguez-Mijangos et al. [2014] was applied to the third lot (UV-C treatment) in a portable disinfection chamber. Groups of three spears were arranged in the center of the equipment at 75 mm from the radiation source for 26 min and 48 s. Approximately 90 g of fresh-cut fruit from each lot were distributed in polyethylene terephthalate (PET) containers (12 cm long, 10 cm wide, and 6 cm high). Fifteen containers per each lot were used for quality analyses. Fresh-cut mango was stored under refrigeration at 5°C with a relative humidity (RH) of 85-90% for 12 days.
Analyses of pH, titratable acidity, total soluble solid content, and firmness
Titratable acidity (TA), pH, total soluble solid (TSS) content, and firmness analyses were performed. pH was measured with a potentiometer (STARTER 2100, OHAUS, Nänikon, Switzerland). TA was assessed by titration with 0.1 N NaOH (Thermo Fisher Scientific, Waltham, MA, USA) considering citric acid as a predominant organic acid in mango. TSS content was determined according to International AOAC 932.12 method using a manual refractometer [AOAC, 2012]. Firmness was measured with a texture analyzer (EZ-S, Shimadzu, Kyoto, Japan) through a Warner-Bratzler shear force test following the methodology proposed by Lázaro & De Lorenzo [2015] with some modifications. The mango samples were cut crosswise with a 2 mm thick Warner-Bratzler steel blade at a test speed of 100 mm/s. Firmness was considered as the maximum force applied to break a piece of mango.
Measurements of color parameters
Mango surface color parameters were evaluated with a MiniScan XE plus portable colorimeter (HunterLab, Reston, VA, USA) in CIELab tristimulus coordinates: L* (lightness: white(+)/black(–)), a* (red(+)/green(–)), and b* (yellow(+)/blue(–)). Equipment was calibrated with a reflector plate (X=80.1, Y=85, Z=90.6) considering a standard illuminant D65 and a 10° observer. Chroma (C*), hue angle (h°), and total color difference (∆E) were calculated with the following formulas [Pathare et al., 2013]:
Microbial count analysis
According to Hinojosa et al. [2013], three randomized samples of 15 g for each treatment were individually homogenized in 135 mL of 0.1% peptone water (Difco, Fisher Scientific, Hampton, NH, USA) in a sterile stomacher bag (Nasco, Whirl-Pak, Madison, WI, USA) for 2 min. Serial dilutions were prepared with 0.1% peptone water. For each dilution, aliquots of 1 and 5 mL were plated in triplicate on different mediums (a total of six Petri dishes per sample). Plates with plate count agar (Difco) and violet red bile agar (BD, Franklin Lakes, NJ, USA) were incubated for approximately 48 h (Analog Incubator 132000, Boekel Scientific, Feasterville, PA, USA) for aerobic mesophilic and total coliform counts, respectively. Plates with plate count agar and potato dextrose agar (Difco) were incubated for 7 days at 5°C and 22°C to count psychrotrophic bacteria and yeast and molds, respectively. The results were expressed as log colony forming units (cfu) per g of fresh-cut mango. The microbial quality of fresh-cut mango was evaluated following Colombian microbial legislation for minimally processed fruits [Ministry of Health and Social Protection of Colombia, 2013].
Inactivation kinetic modelling
A model of inactivation of microorganisms was developed using the linear logarithmic equation and Weibull model as a function of storage days [van Boekel, 2002] using formulas (4) and (5), respectively.
where: N0 and N are the numbers of initial and surviving microorganisms, respectively, and t corresponds to time of storage (day), k is the inactivation rate constant (1/day), α is the characteristic time (day), and β is the shape factor.The inactivation parameters were estimated with the averages of the logarithmic reductions of the microbial populations during the storage time. Linear least squares method and non-linear least squares method with the resolution of the Gauss-Newton algorithm (maximum number of iterations of 200 and a convergence tolerance of 0.00001) were used to fit the inactivation data of microorganisms to the logarithmic linear equation and to the Weibull model, respectively. The fit of the models was evaluated by calculating the coefficient of determination (R2), the adjusted coefficient of determination (adjusted R2), and the root mean square error (RMSE).
Extract preparation
Two grams of mango samples were added to 3 mL of absolute ethanol. The solutions were mixed (MX-S vortex mixer, Science Med, Helsinki, Finland), sonicated for 30 min (Branson 1800 Digital Bath, Emerson, St. Louis, MO, USA), and placed in refrigeration and darkness for night. Subsequently, these solutions were centrifuged at 257×g for 20 min (Compact II centrifuge, BD). The supernatant was used for the quantification of bioactive compounds.
Total carotenoid content determination
Following the method proposed by González-Vega et al. [2021], 300 μL of each extract were added in triplicate to wells of the microplate, and absorbance was measured at 450 nm in a 96-well microplate reader (Multiskan GO, Thermo Fisher Scientific). A calibration curve was plotted with concentrations between 0.002 and 0.1 mg/mL of β-carotene standard (Sigma-Aldrich, St. Louis, MO, USA) (R2=0.991). Results were reported as mg β-carotene equivalents per 100 g of fresh weight (FW) of mango.
Determination of total phenolic and flavonoid contents
The total contents of phenolics and flavonoids were determined following Pérez-Perez et al. [2021] methodology. For total phenolic content, 10 μL of each extract and 25 μL of 0.2 M Folin-Ciocâlteu reagent solution (Sigma-Aldrich) were initially added to each well of the microplate. The mixture was left under refrigeration for 5 min and subsequently, 25 μL of 20% Na2CO3 and 140 μL of distilled water were added. The microplate was put to rest for 30 min, and the absorbance was measured at 760 nm on the microplate reader. A calibration curve was plotted with concentrations between 0.1 and 1 mg/mL of gallic acid standard (Sigma-Aldrich) (R2=0.992). The results were expressed as mg gallic acid equivalents (GAE) per 100 g of FW of mango.
For total flavonoid content determination, 80 μL of each extract and 80 μL of an ethanolic solution of aluminum trichloride (20 g/L) were added to wells of the microplate. The microplate was shaken for 30 s and left in the dark for 1 h at 25°C. Subsequently, it was shaken once more for 30 s, and the absorbance was measured at 415 nm in the microplate reader. The calibration curve was plotted with the quercetin standard (Sigma-Aldrich), considering concentrations from 0 to 0.08 mg/mL (R2=0.999). The results were reported as mg quercetin equivalents (QE) per 100 g of FW of mango.
Antioxidant capacity assays
Assays were performed following the methodology reported by Pérez-Perez et al. [2021] with some modifications. To determine the antioxidant capacity by scavenging 2,2-diphenyl-1-picrylhydrazyl (DPPH) radical, 1 mg of the DPPH radical (Sigma-Aldrich) was weighed and dissolved in 1 mL of absolute ethanol. From this initial solution, 0.75 mL was added to 29.25 mL of ethanol. The absorbance of the resulting solution was adjusted to 0.7 at a wavelength of 515 nm. Next, 200 μL of the resulting solution and 20 μL of the extract were added to wells of the microplate. The absorbance was measured at 515 nm in the plate reader after 30 min of leaving the microplate to rest. A calibration curve was made with Trolox standard (Sigma-Aldrich) considering concentrations between 0 and 0.2 mg/mL (R2=0.992). The results were expressed as mM Trolox equivalents (TE) per g of FW of mango.
For determination of the antioxidant capacity by 2,2’-azino-bis(3-ethylbenzothiazoline-6-sulfonic acid) (ABTS) assay, 19.3 mg of ABTS were dissolved in 5 mL of distilled water. Separately, 0.0378 g of potassium persulfate and 1 mL of distilled water were mixed. From the second solution, 88 μL were added to the first solution, and it was left to rest in the dark for 16 h under refrigeration. One mL of this last prepared solution containing generated ABTS radical cations was added to 88 mL of ethanol, and the absorbance was adjusted to 0.7 at a wavelength of 734 nm. For the measurement of the samples, 270 μL of the ABTS radical cation solution and 20 μL of each extract were taken. The absorbance was measured at 734 nm after 30 min of rest. The calibration curve was plotted with Trolox standard considering concentrations from 0 to 0.01 mg/mL (R2=0.994). The results were expressed as mM TE/g FW.
For ferric reducing antioxidant power (FRAP) determination, the stock solutions were prepared: a 300 mM sodium acetate buffer at pH 3.6, a 20 mM FeCl3×6H2O solution, and a solution of 10 mM 2,4,6-tris(2-pyridyl)-s-triazine (TPTZ; Sigma-Aldrich) in 40 mM HCl. The working solution was obtained by adding the stock solutions in a 10:1:1 (v/v/v) ratio (buffer, FeCl3×6H2O, and TPTZ-HCl, respectively). Then, 280 μL of the working solution and 20 μL of each extract were added to each well of the microplate. Absorbance was measured at 638 nm after 30 min of rest. The calibration curve was plotted with Trolox standard considering concentrations between 0 and 0.4 mg/mL (R2=0.999). Results were reported as mM TE/g FW.
Statistical analysis
A completely randomized design with two factors and a 3×5 factorial arrangement was used. The first factor (A) was the treatment of disinfection of fresh-cut mango with three levels (without disinfection treatment or control (C), immersion in 10 mg/L sodium hypochlorite solution (H), and application of a UV-C dose of 6 kJ/m2 (UV)). The second factor (B) corresponded to the sampling time during storage of fresh-cut mango in refrigeration, comprising five levels (0, 3, 6, 9, and 12 days). Fresh-cut mango from the control treatment was considered as a negative control, and from the treatment with NaClO as a positive control. Results were presented as the mean of three replicates. A two-way ANOVA and a Tukey’s test were performed to compare mean values between treatments and evaluate the effect of factors on pH, TA, TSS content, firmness, color parameters, microbial counts, contents of total carotenoids, total phenolics and flavonoids, and antioxidant capacity of fresh-cut mango (p<0.05).
RESULTS AND DISCUSSION
Titratable acidity, pH, total soluble solid content, and firmness
The results of determinations of titratable acidity, pH, total soluble solid content, and firmness of mango treated with UV-C, immersed in sodium hypochlorite solution and without treatment during storage are shown in Figure 1. According to the two-way ANOVA, the disinfection treatment, the storage time, and the interaction between these factors had a significant effect on the physicochemical properties of fresh-cut mango (p<0.05). Values of pH indicated that fresh-cut mango from C (3.88–4.18) and H (3.75–3.95) treatments was entering the senescence stage. For mango irradiated with UV-C, pH started to decrease significantly since day 9 of storage. According to Zambrano-Zaragoza et al. [2021], UV-C treatment allows delaying senescence stage. The content of TSS of fresh-cut mango from UV treatment tended to increase until day 9 of storage. The increase in TSS content is attributed to the hydrolysis of polysaccharides and the accumulation of released soluble sugars as a result of fruit ripening during storage [George et al., 2015; Khubone & Mditshwa, 2018]. On the other hand, TSS content of C sample remained almost constant and its content of H sample decreased slightly only at day 6 (14.13 °Brix) of storage (Figure 1). Treatments could affect the rate of transpiration, generating slight changes in the content of water in the fruit [Zambrano-Zaragoza et al., 2021]. The titratable acidity of fresh-cut mango from C treatment exhibited an increase up to day 9 of storage (0.48–0.85 g/100 g) and then decreased slightly (to 0.76 g/100 g) (Figure 1). The reduction in acidity levels can be initially linked to the ripening of the fruit when organic acids are degraded [Barreto et al., 2021]. For the samples from H and UV treatments, TA increased until day 3 of storage and then remained almost constant (Figure 1). The disinfection treatments and the modified atmosphere could slow down the respiration rate, and the utilization rate of the respiratory substrate was minimal through the storage period [Razali et al., 2021].
Figure 1
Values of pH, total soluble solid (TSS) content, titratable acidity (TA), and firmness of fresh-cut mango without treatment (control, C), immersed in sodium hypochlorite solution (H), and treated with ultraviolet short wave (UV-C, UV) during storage at 5°C for 12 days. Vertical lines above and below the points correspond to standard error. Different lowercase letters (a-d) above bars (separately for control and each treatment) indicate significant differences (p<0.05).
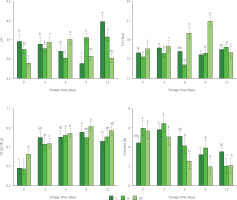
The firmness of fresh-cut mango tended to decrease during refrigerated storage (Figure 1). According to Barreto et al. [2021], the reduction of firmness of minimally processed fruits is generated by the cutting operation and the high degree of injury to cellular tissues. The demethylation and depolymerization of pectin, generation of free radicals, enzymatic degradation of hemicellulose, solubilization of polyuronides, the release of galactose from pectic polymers, cell wall swelling, decreased water content and osmotic potential may occur in tissues and thus lower the fruit firmness [Barreto et al., 2021; Márquez-Villacorta & Pretell-Vásquez, 2013]. In our study, it was found that mango irradiated with UV-C exhibited the lowest firmness for most of the storage time (2.10–5.63 N) compared to control (3.53–4.46 N) and H-treated (2.03–5.94 N) samples (Figure 1). Fruit softening is modulated by cell wall hydrolases, such as polygalacturonase, cellulase, β-galactose, and expansin, and UV-C treatment can alter the expression of genes involved in modifying cell wall structure [Kan et al., 2021].
Color parameters
It was found that the disinfection treatment had no significant effect on L* coordinate of fresh-cut mango (p≥0.05). However, the storage time and the interaction of the factors had a significant effect on the values of this parameter (p<0.05). In Table 1, it is shown that L* color coordinate of mango samples from C and UV treatments tended to increase slightly during storage, and to decrease for the samples from H treatment. Luminosity is considered an indicator of browning. The L* value of the H-treated fresh-cut mango decreased during the storage possibly due to sample browning as a result of oxidation processes induced by NaClO. The disinfectants served as oxidizing agents [Hinojosa et al., 2013]. In the case of UV-C treatment, inhibition of polyphenol oxidase (PPO) activity by radiation could occur [Barreto et al., 2021] and result in the reduced browning of the fruits.
Table 1
Color parameters of fresh-cut mango without treatment (control, C), immersed in sodium hypochlorite solution (H), and treated with ultraviolet short wave (UV-C, UV) during storage at 5°C for 12 days.
The disinfection treatment, the storage time, and the interaction between these factors had a significant effect on all other color parameters of fresh-cut mango (p<0.05). Low values of a* coordinate represented tones between green and red. The values of a* increased for the control sample, decreased for the samples from H treatment, and remained slightly constant for the samples from UV treatment during storage (Table 1). The increase in a* coordinate value can be interpreted as the increase in orange or red pigments due to fruit ripening, and on the other hand, as enzymatic and non-enzymatic browning [Márquez-Villacorta & Pretell-Vásquez, 2013; Nguyen et al., 2022]. Therefore, the radiation treatment allowed delaying some adverse processes linked to accelerated ripening and browning of the fruit, which possibly occurred in mango from C and H treatments, respectively. Positive values of b* coordinate indicated yellow tones. This coordinate tended to remain constant for the samples from H and UV treatments during storage (Table 1). During mango ripening, green chloroplasts become chromoplasts with red and yellow tones. These tones in mango are mainly derived from β-carotene, which is accumulated in the chromoplasts in the form of crystalloids [Miguel et al., 2016]. For the control mango, b* coordinate value increased throughout cold storage (Table 1). According to Rosalie et al. [2018], low temperatures can delay or block the accumulation of carotenoids in fruits, since it promotes oxidation in tissues.
The C* value increased during storage for the control sample and tended to remain constant for the samples from H and UV treatments (Table 1). The increase in C* value for the control sample indicated that the color became more saturated due to storage. This could stem from the appearance of some brown tones [Grasso et al., 2022]. Values of h° increased significantly (p<0.05) after day 9 of storage for mango from H treatment. It can be attributed to the accelerated isomerization of carotenoids and oxidation by free radicals [Xiang et al., 2020]. In turn, the h° values tended to decrease slightly for the control (from 81.52 to 77.46) and UV-C (from 81.62 to 80.24) treated samples throughout the storage (Table 1). A decrease in h° values indicates the yellowing of the fruit [Barreto et al., 2021], while delay in color development is linked to a reduction in ripening and senescence [Khubone & Mditshwa, 2018]. According to the classification proposed in literature [Pathare et al., 2013], the total color differences (ΔE) of the stored samples (relative to storage day 0) in our study were mostly classified as very different (ΔE>3) and different (1.5<ΔE<3). The ΔE increased significantly (p<0.05) throughout the storage of the control and H-treated samples. The UV-C-treated sample showed the least color change (the lowest ΔE value) after 12 days of storage. The irradiation of fresh-cut mango allowed to prevent the oxidation reactions associated with the activity of peroxidase (POD) and stabilize the pigments and color parameters [Zambrano-Zaragoza et al., 2021].
Results of microbiological analysis
Figure 2 shows a significant increase in the native microbial population of fruit during storage. This increase may have been due to the handling of the samples during cutting and the high surface area/weight ratio of each sample [Artés-Hernández et al., 2021]. By the two-way ANOVA, it was found that the disinfection treatment, the storage time, and their interaction had a significant effect on the native microorganisms of fresh-cut mango (p<0.05). The initial population of aerobic mesophiles was 1.69, 1.66, and 1.42 log cfu/g for the samples from C, H and UV treatments, respectively (Figure 2). This population increased notably for the samples from C and H treatments during the storage time. For UV-C irradiated samples, it remained stable after day 6 of storage. The H and UV treatments reduced the population of aerobic mesophiles of mango by 0.26 and 0.62 log cfu/g, respectively, compared to C treatment at day 12 of refrigerated storage. For total coliforms, the initial population was 1.90, 2.23, and 2.08 log cfu/g for fresh-cut mango from C, H, and UV treatments, respectively. Total coliform count of the samples tended to increase during storage. At the end of storage, the treatments with disinfectant agents promoted a reduction of about 0.5 log cfu/g of enterobacteria population in contrast to C treatment. The initial population of psychrophiles was 1.47, 1.45, and 1.32 log cfu/g for fresh-cut mango from C, H, and UV treatments, respectively. Psychrotropic bacteria increased during storage in all samples. At the end of storage, the population reduction in the samples from H and UV treatments was 0.18 and 0.32 log cfu/g, respectively, in contrast to samples from C treatment.
Figure 2
Aerobic mesophilic, total coliforms, yeast and molds, and psychrotrophic populations on fresh-cut mango without treatment (control, C), immersed in sodium hypochlorite solution (H), and treated with ultraviolet short wave (UV-C, UV) during storage at 5°C for 12 days. Vertical lines above and below the points correspond to standard error. Different lowercase letters (a-e) above bars (separately for control and each treatment) indicate significant differences (p<0.05).
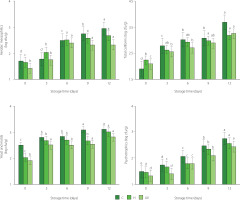
According to Colombian microbiological requirements, the population of molds and yeasts limited the quality of fresh-cut mango (3 log cfu/g) [Ministry of Health and Social Protection of Colombia, 2013]. The samples from C and H treatments presented an acceptable level of quality until day 3 and 9 of storage, respectively (Figure 2). While the UV-treated samples had an acceptable level of quality throughout storage.
For kinetics inactivation (Table 2), Weibull model explained the inactivation of almost all native microorganisms of fresh-cut mango from different treatments. According to van Boekel [2002], the shape parameter (β) value lower than 1 indicated that some populations of native microorganisms had the ability to adapt to the stress applied with the treatments. While β>1 meant that in other populations, there remained cells that were damaged by the applied stress.
Table 2
Fitted and inactivation kinetic parameters of native microorganisms of fresh-cut mango without treatment (control, C), immersed in sodium hypochlorite solution (H), and treated with ultraviolet short wave (UV-C, UV) during storage at 5°C for 12 days.
Bioactive compound contents
The disinfection treatment, the storage time, and their interaction had a significant effect on the total carotenoid and total flavonoid contents of fresh-cut mango (p<0.05). In turn, storage time had no effect on the total phenolic content of fresh-cut mango (p≥0.05). The content of total carotenoids was 5.77, 11.42, and 20.95 mg β-carotene/100 g FW for C, H, and UV samples at the beginning of storage, respectively, and 19.34, 19.04, and 26.50 mg β-carotene/100 g FW at 12 day of storage, respectively, with maxima on day 3 of storage for the control and H-treated mango, and on day 6 of storage for the fruit from UV-C treatment (Figure 3). Accumulation of carotenoids during the storage could be influenced by the maturity of the fruit [Rosalie et al., 2018]. Samples from control and H treatments which showed accelerated ripening and browning could reduce the accumulation of carotenoids due to metabolic processes. UV-C irradiation influenced carotenoid content due to physiological stress. UVR8 protein acts as a photoreceptor and interacts with another encoding protein COP1 to stimulate the production of carotenoids as a photoprotective effect [Castillejo et al., 2022]. The increase in the content of total carotenoids is also related to the activation of carotene isomerase enzyme and the accumulation of phytoene synthase (PSY) and zeta-carotene desaturase (ZDS) gene transcripts [Khubone & Mditshwa, 2018].
Figure 3
Contents of total carotenoids, total phenolics, and flavonoids of fresh-cut mango without treatment (control, C), immersed in sodium hypochlorite solution (H), and treated with ultraviolet short wave (UV-C, UV) during storage at 5°C for 12 days. Vertical lines above and below the points correspond to standard error. Different lowercase letters (a-d) above bars (separatly for control and each treatment) indicate significant differences (p<0.05). GAE, gallic acid equivalents; QE, quercetin equivalents; FW, fresh weight.
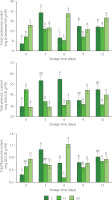
The total phenolic content of fresh-cut mango of control and UV-C treated fruit was higher than that of the H samples at 6, 9, and 12 day of storage (Figure 3). This variation in the total phenolic content may be attributed to the various reasons. The release of phenolic compounds bound to cell wall polysaccharides and the depolymerization of phenolic polymers such as tannins [Xiang et al., 2020] might have occurred. On the other hand, phenolics can be used as substrates for enzymes such as β-glucosidase, PPO, and POD [Velderrain-Rodríguez et al., 2021]. These processes may occur to varying degrees in mango samples subjected to various treatments and thus result in different total phenolic content.
Fresh-cut mango showed a low content of flavonoids, which could be due to the low expression of the flavonol synthase in the ripening of the fruit, although the flavonol glycoside (quercetin 3-galactoside) is the major flavonoid of mango (22.1 mg/kg) [Maldonado-Celis et al., 2019]. In our study, there were maxima in flavonoid content at days 3 (1.00 mg QE/100 g FW) and 6 (1.14 mg QE/100 g FW) of storage for the samples from C and UV treatments, respectively (Figure 3). The UV-C irradiation leads to the regulation of oxidative stress and the biosynthesis of key enzymes in the flavonoid biosynthetic pathway, such as phenylalanine ammonium lyase (PAL) and chalcone synthase (CHS) [Michailidis et al., 2019]. The stress caused by UV-C can increase the production of reactive oxygen species (ROS), ROS scavenging compounds, and absorbers of UV radiation [Castillejo et al., 2022]. UV-C stress can also activate the signaling pathway of the COP1 and UVR8 photoreception proteins to produce flavonoids [Li et al., 2017].
Antioxidant capacity
It was established that the disinfection treatment, the storage time, and the interaction between the factors had significant effects on the antioxidant capacity of fresh-cut mango (p<0.05). Except that the storage time had no significant effect on the antioxidant capacity measured by DPPH assay of fresh-cut mango (p≥0.05). At the end of storage, DPPH• scavenging activity of the control (0.27 mM TE/g FW) was lower compared to both treated samples. For the fresh-cut mango treated with UV-C, DPPH• scavenging activity was the highest (0.60 mM TE/g of FW). It could be due to the increase in the activity of PAL in response to external stress caused by fruit irradiation [Prajapati et al., 2021].
The antioxidant capacity measured by ABTS assay tended to remain constant for the control sample (0.56–0.61 mM TE/g FW), and to increase for the samples from H treatment (0.39–0.56 mM TE/g FW) throughout storage (Figure 4). In turn, the activity of the samples treated with UV-C remained almost constant from day 0 to day 9 (0.58–0.62 mM TE/g FW). It is possible that UV-C affected the integrity of the cell structure and vacuoles, promoting the release of compounds with antioxidant activity [Rybak et al., 2021]. At the end of the storage, ABTS•+ scavenging activity of the UV-C treated samples decreased (0.48 mM TE/g FW). Miguel et al. [2016] reported that the high activity of PPO and the low activity of PAL in mango during the beginning of senescence have an impact on its ABTS•+ scavenging activity.
Figure 4
Antioxidant capacity determined as DPPH• and ABTS•+ scavenging activities and as ferric-reducing antioxidant power (FRAP) of fresh-cut mango without treatment (control, C), immersed in sodium hypochlorite solution (H), and treated with ultraviolet short wave (UV-C, UV) during storage at 5°C for 12 days. Vertical lines above and below the points correspond to standard error. Different lowercase letters (a-c) above bars (separatly for control and each treatment) indicate significant differences (p<0.05). TE, Trolox equivalents; FW, fresh weight.
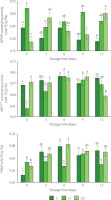
The FRAP exhibited slight maxima on day 6 of storage for mango spears from C (0.68 mM TE/g FW) and UV (0.69 mM TE/g FW) treatments, and on day 9 of storage for the samples from H treatment (0.64 mM TE/g FW) (Figure 4). Low refrigeration temperature and UV-C irradiation stress activated the defense mechanism of enzymes related to the elimination of ROS and the biosynthesis of antioxidant compounds [Hinojosa et al., 2013].
CONCLUSIONS
The UV-C treatment of fresh-cut ‘Tommy Atkins’ mango proved effective in preserving its quality attributes. The application of a UV-C dose of 6 kJ/m2 promoted the conservation of microbial safety, the physicochemical properties (pH, TSS, and AT), the color parameters (L*, a*, and b*), the contents of total carotenoids, total phenolics, total flavonoids, and the antioxidant capacity measured by DPPH assay and as FRAP during fruit storage for 12 days at 5°C. However, this treatment promoted the loss of fruit firmness. In general, the disinfection treatment of fresh-cut mango with UV-C is a technological alternative to replace the conventional disinfection method with sodium hypochlorite immersion, since it provides the conservation of the quality attributes of fruit, mitigates the adverse impact on the environment and on the health of consumers by the lack of dangerous chemicals applied during minimal processing.