INTRODUCTION
Nanguo pear (Pyrus ussuriensis Maxim.) belongs to Qiuzi pear series and is one of the characteristic fruits in Liaoning province, China [Tao et al., 2019]. Fruits of this plant are sweet and sour with a rich and unique aroma [Wei et al., 2017], which is deeply loved by consumers. In China, these pears are not only eaten as delicious food, but also considered as a cough medicine and diuretic [Cui et al., 2005]. They contain ascorbic acid and phenolic compounds, including arbutin, catechin, chlorogenic acid, quercetin, and rutin, that determine their biological activities, such as antioxidant and anti-inflammatory effects [Chen et al., 2007; Li et al., 2012; Yan et al., 2023].
Nanguo pear is a respiratory climacteric fruit with a relatively short shelf life [Wang et al., 2017]. The ripening and senescence of the fruit can be appropriately delayed when stored at low temperature, but the browning of the peel occurs easily when the Nanguo pear is brought to room temperature [Zhang et al., 2018]. Freezing is one of the most important long-term storage methods for food, which allows consumers to buy seasonal fruit throughout the year. However, in the process of storage, transportation and consumer processing, frozen food is prone to temperature fluctuations, resulting in freeze-thaw cycles, and food may suffer mechanical damage, nutrient denaturation and loss of water retention ability [Ndraha et al., 2018].
The flavor of fruit is an important characteristic to evaluate its quality, and is closely related to the content of carbohydrates, organic acids and volatile organic compounds [Aprea et al., 2017; Cui et al., 2022]. Luo et al. [2021] reported that the contents of volatile esters in Nanguo pears peaked during the optimum taste period (OTP) and that especially ethyl butyrate, ethyl caproate and hexyl acetate dominated in the profile of these compounds. Other research also shows that these aroma compounds of Nanguo pear have the advantages of high content and low odor threshold [Zhang & Yin, 2023].
The main purpose of this study was to determine the effects of multiple freeze-thaw cycles on Nanguo pear flavor compounds. Gas chromatography-mass spectrometry (GC-MS) method was used to measure the changes in main volatile esters in Nanguo pear, and electronic nose (e-nose) and electronic tongue (e-tongue) were deployed to comprehensively analyze the differences in the flavor of Nanguo pear. The content of acids and sugars in pears was determined as well, so as to explore the change of Nanguo pear flavor compounds in the freeze-thaw cycles.
MATERIALS AND METHODS
Materials and chemicals
Mature pear fruits (variety Nanguo) were hand-harvested from the local commercial orchard in Jinzhou, Liaoning Province, China. The collected material (about 65 kg) was transported to the laboratory of the Bohai University in Jinzhou, Liaoning Province, China, and refrigerated at 4°C in a low-temperature constant incubator (Sanyo MIR 254, Sanyo Electric Co., Ltd, Osaka, Japan) for 24 h before experiments.
Freeze-thaw cycles
The refrigerated Nanguo pears were randomly equally divided into 6 groups, 10 kg in each group. In addition, 5 kg was used as a fresh sample. Fruits of experimental groups denoted by the symbols SF – slow freezing (3 groups) and FF – fast freezing (3 groups) were frozen at −20°C and −80°C, respectively, in a refrigerating machine for 24 h. After freezing, the pears were transferred to a low-temperature constant incubator for thawing, and then continued to freeze. This freezing and thawing process was repeated 1, 2 and 3 times at each freezing temperature to obtain the samples with different freeze-thaw cycles (FT1, FT2, FT3, respectively).
Determination of the content of total soluble solids
Randomly selected 10 Nanguo pears were peeled and cut into pieces, then beaten into a pulp and filtered with 300-mesh filter cloth, and the filtrate was dropped into a digital refractometer (PAL-3 hand-held sugar meter, ATAGO Co., Ltd, Tokyo, Japan) for determination of the content of total soluble solids (TSS), which was expressed as a g/100 g.
Determination of organic acid content
Referring to the method of Zhang et al. [2021], high performance liquid chromatography (HPLC), using Shimadzu LC2030 system equipped with a UV detector (Shimadzu Corp., Kyoto, Japan), was applied to determine the content of organic acids. The thawed Nanguo pear flesh tissue (5.0 g), was placed into a spiral-cap centrifuge tube (50 mL). An aliquot of 25 mL of pure water was added into the test tube, and ultrasonic extraction was performed for 30 min in an ultrasonic bath (KQ-500DE ultrasonic cleaner, Kunshan Ultrasonic Instrument Co., Ltd, Kunshan, Jiangsu Province, China) at 80°C. Then, the mixture was cooled at room temperature and centrifuged at 12,000×g at 4°C for 20 min in the Biofuge Stratos centrifuge (Thermo Scientific, Waltham, MA, USA). After the supernatant was poured out, the purified water was added again to repeat the previous operations. Finally, both supernatants were mixed, and the volume was filled to 50 mL. The extract prepared in this way was filtered through a 0.45 μm syringe, put into a vial, and stored at low temperature until analyzed.
An Eclipse Plus C 18 column (particle size 5 μm, 250×4.6 mm, Agilent, Santa Clara, CA, USA) was used to separate organic acids by HPLC. The mobile phase consisted of methanol (5.0% of the mobile phase volume, v/v) and 0.01 mM dipotassium hydrogen phosphate aqueous solution (95% of the mobile phase volume, v/v). The isocratic elution was carried out with the mobile phase flow rate of 0.5 mL/min. The injection volume was 10 μL, the detection wavelength was set to 210 nm, and the column temperature was maintained at 30°C. Under the same conditions, the standard curve was made from the standards (HPLC grade), including oxalic acid, l-tartaric acid, l-malic acid, shikimic acid and citric acid, which were purchased from Beijing Solaibao Technology Co., Ltd, China. The content of organic acids in pear flesh was expressed as μg/g.
Odor analysis using electronic nose
The odor of the pears was analyzed using a portable electronic nose (PEN3 e-nose, Airesense Analytics GmbH, Schwerin, Germany). The e-nose consisted of a sampling system, a unit with metal oxide sensors, and pattern recognition software (Win-Muster 1.6.2.15/May 17, Airsense Analytics GmbH). The response characteristics of each sensor were shown as follows: R1 – aromatic compounds, R2 – nitrogen oxide, R3 – ammonia and aromatic compounds, R4 – hydrogen, R5 – olefin and aromatic compounds, R6 – hydrocarbons, R7 – hydrogen sulfide, R8 – alcohols and partially aromatic compounds, R9 – aromatic compounds and organic sulfides, and R10 – alkanes [Zhu et al., 2020]. The thawed pears were cut into chunks and pulped, then filtered through 300-mesh filter cloth. An aliquot of 15 mL of the filtrate was placed in a 50 mL plastic pipe, which was sealed with three layers of plastic wrap. The sample was left standing for 30 min until the odorous substances accumulated above the liquid. The cleaning time of the e-nose was 100 s, the measurement phase lasted 120 s, and the detection environment was kept at 25°C. To minimize error, the surrounding environment was kept closed during measurement.
Taste analysis using electronic tongue
Taste analysis of the samples was performed with an SA402B electronic tongue (Insent/Intelligent Sensor Technology, Inc., Kanagawa, Japan), which could test up to 10 samples at a time. The measuring cup containing samples, positive and negative electrode liquid and reference liquid were placed in the sample tanks, respectively [Zhu et al., 2020]. The positive electrode liquid contained 30% (v/v) ethanol, 100 mM KCl and 10 mM KOH; the negative electrode liquid contained 30% (v/v) ethanol and 100 mM HCl; and the reference liquid consisted of 30 mM KCl and 0.3 mM tartaric acid. Before testing, the thawed pears were homogenized and filtered, and then centrifuged. The supernatant was 10-fold diluted, then 70 mL of the solution was poured into two 50 mL sample cups. Before starting the measurement, the sensor was inserted for 30 s, then the measurement was performed for another 30 s. The sensor was cleaned automatically after the measurement, and the measurement was repeated four times for each sample. The first measurement cycle was discarded due to instability, and only the later three stable sensor responses were used as the raw data for the sample.
Determination of volatile ester profile
Analysis of pear volatile esters was performed using 7890N/5975 GC-MS instrument (Agilent). A portion of 3.0 g of thawed pear flesh was weighed into a 20 mL sample bottle, 5 mL saturated NaCl solution was added, and then 20 µL cyclohexanone solution with a concentration of 0.475 mg/mL was added as the internal standard [Zhou, 2015]. Two magnetic rosters were put into each sample bottle, and the headspace bottle was sealed. After the closed headspace bottle was shaken well, it was balanced in the magnetic agitator at 45°C for 10 min. Then, the needle handle was pushed to stretch out the extraction head and adsorbed for 30 min. After the adsorption, the extraction head was quickly recovered. The HP-5MS capillary column (30 m×0.25 mm×0.25 µm; Agilent) was used to separate volatile compounds. The sample inlet temperature and the ion source temperature were set at 250°C and 230°C, respectively. The column temperature was 40°C and the quadrupole was maintained at 150°C. Helium gas was used as a carrier gas, and its flow rate was 1.2 mL/min. The programmed heating process was as follows: the initial column temperature was set at 40°C for 5 min, and then it was increased to 120°C at the rate of 4°C/min, and kept for 2 min to complete the first heating stage. Then, the temperature was increased to 230°C at a rate of 10°C/min and kept for 6 min to complete the second heating stage. The results were matched with the mass spectrum library data to identify the volatile esters. The peak area of cyclohexanone was used to calculate the content of identified compounds in pear flesh, which was expressed as µg/kg.
Statistical analysis
The experiment was performed three times with three replicates each. The data were statistically processed using the SPSS17.0 (SPSS Inc., Chicago, IL, USA). One-way analysis of variance (ANOVA) with least significant difference (LSD) and Duncan tests were used to compare the samples. The differences at p<0.05 were considered statistically significant. Principal component analysis (PCA) and Linear discriminant analysis (LDA) were performed on the e-nose data using Win-Muster software. Origin 9.5 (OriginLab Corporation, Northampton, MA, USA) was used to plot the changes of each analyzed index.
RESULTS AND DISCUSSION
Effects of freeze-thaw cycles on the content of total soluble solids of Nanguo pear
Total soluble solid (TSS) is a very important characteristic for the sensory and nutritional quality of food [Keutgen & Pawelzik, 2007a,b; Vu et al., 2023]. TSS of fruits usually refer to soluble sugars, such as glucose and fructose, which reflects the sweetness of fruits [Kanski et al., 2020]. The content of TSS of Nanguo pear fruit after each freeze-thaw cycle is shown in Figure 1. The fresh pears had TSS content of 14.17 g/100 g. This value was similar to that previously reported by Yan et al. [2023] for fresh Nanguo pear (14.70 g/100 g), but higher than the contents of 13.4 g/100 g and 8.9 g/100 g reported by Wang et al. [2021] and Chen et al. [2007], respectively, which may be due to the different origin of the fruit (different growing conditions, different variety). The content of TSS in the freeze-thaw samples was significantly (p<0.05) higher than that in fresh fruits. After the first freeze-thaw cycle, the content of TSS in pears frozen at −20°C was 17.57 g/100 g, and that in fruits of FF-FT1 group was 18.17 g/100 g. Ice crystals formed during the freezing lead to destruction of cell structures and damage to cell walls. The rise in TSS content of pears after freezing may be caused by the destruction of vacuoles by ice crystals and the massive outflow of cell fluid. Repeated freezing and thawing will cause more water loss, which will reduce the TSS content. However, there was a study speculating that the degradation of starch and other substances caused by long-term storage increases the content of soluble sugars [Liu et al., 2019], which explains why the TSS content has increased after three freeze-thaw cycles. Some studies had also pointed out that fructose and glucose contents of slow frozen (−20°C) Prunus mume fruits were higher after thawing than of the fast frozen fruits (−50°C) [Chung et al., 2013]. With subsequent freeze-thaw cycles under the two freezing conditions, the content of TSS first decreased and then increased (Figure 1). However, under the same number of freeze-thaw cycles, there was a significant (p<0.05) difference in the content of TSS under the two freezing conditions, indicating that the content of TSS was related to the freezing method.
Effects of freeze-thaw cycles on organic acid content of Nanguo pear
The composition of organic acids is an important factor influencing the flavor quality and nutritional value of fruits. In addition, organic acids can provide energy for the body, prevent cardiovascular disease by regulating metabolism, while showing excellent bacterial-inhibiting function [Shi et al., 2022].
In organic acid composition of fresh Nanguo pears, the content of citric acid reached 63.50 µg/g and was significantly (p<0.05) higher than those of other acids (Table 1). In other studies, Wang et al. [2021] determined the content of citric acid in fresh Nanguo pear at 1.4 mg/g and Chen et al. [2007] at 309 mg/kg. These values are different from the results determined in this study, which may be due to differences in the maturity of the purchased pears. The content of citric acid decreased after one freeze-thaw cycle to 38.26 and 32.17 μg/g in both slow (−20°C) and fast (−80°C) freezing conditions, respectively. The opposite phenomenon was observed by Liu et al. [2019], who found that the citric acid content in fresh ’Ruane’ pears and these pears frozen at −20°C and directly thawed was similar (p<0.05), and even increased when the frozen fruits were stored for 1–12 days. The content of citric acid in pears of FF-FT2 and FF-FT3 groups was lower than that in SF-FT2 and SF-FT3 groups, respectively (Table 1). Contents of oxalic acid, shikimic acid, l-tartaric acid and l-malic acid in pears were also significantly (p<0.05) lower after freeze-thaw cycles compared to fresh fruits. The total amount of five organic acids decreased subsequently after each freeze-thaw cycle, and pears of the FF-FT1 group had slightly higher total organic acid content than the fruits from the other treatment groups. The sum of organic acids in the SF-FT3 group and the FF-FT3 group was very similar, indicating that freezing conditions had no significant effect on the total organic acid content of Nanguo pears when the number of freeze-thaw cycles was high.
Table 1
The content of organic acids (µg/g flesh) in fresh Nanguo pears and after three freeze-thaw cycles (FT1, FT2 and FT3, respectively) with freezing at −20°C (SF) and −80°C (FF).
Effects of freeze-thaw cycles on the odor of Nanguo pear
An electronic nose system is a kind of odor-testing equipment, replacing the human olfactory system, with higher sensitivity and faster detection speed [Zhang et al., 2008]. In Figure 2A, the radar chart is shown established by the e-nose sensor data collection according to the response value of each sample, and each curve represents the odor intensity of the fresh pears and pears after each freeze-thaw cycle. There were no differences (p≥0.05) between the samples in the response values of sensors R4, R7 and R9, e.g., to hydrogen, inorganic sulfides such as hydrogen sulfide, and organic sulfides. However, there were significant differences (p<0.05) in sensor R2 and R6 response, indicating that multiple freeze-thaw treatments had the greatest effect on nitrogen oxide and methyl-containing hydrocarbons in pears. The response values of other sensors were also slightly different between samples. These differences might be due to the release of volatile compounds due to the rupture of cell membranes caused by ice crystals during repeated freeze-thaw cycles [Pedrosa-López et al., 2022]. The radar map showed no significant differences between the fruit samples subjected to rapid freezing and slow freezing.
Figure 2
Results of odor analysis by e-nose of Nanguo pear after three freeze-thaw cycles (FT1, FT2 and FT3, respectively). (A) Radar diagram of response value of R1–R10 e-nose sensors (R1 – aromatic compounds, R2 – nitrogen oxide, R3 – ammonia and aromatic compounds, R4 – hydrogen, R5 – olefin and aromatic compounds, R6 – hydrocarbons, R7 – hydrogen sulfide, R8 – alcohols and partially aromatic compounds, R9 – aromatic compounds and organic sulfides, R10 – alkanes); (B) Principal component analysis (PCA) plot; (C) Linear discriminant analysis (LDA) plot. SF means freezing at −20°C and FF means freezing at −80°C.
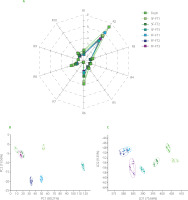
Figure 2B shows the principal component analysis (PCA) diagram of Nanguo pears after 1, 2 and 3 freeze-thaw cycles under different freezing conditions, using the response values of e-nose sensors as variables. The first principal component (PC1) represented 80.21% of total variability, and the second principal component (PC2) – 19.56%. The explanatory rate of total variability was 99.77%, greater than 70∼85%, indicating that these two factors held most of the sample information [Chen et al., 2021]. There was significant discrimination along PC1 between the fresh Nanguo pear and the freeze-thaw Nanguo pears, indicating that there were differences in their odor substances. Variables for the SF-FT2 and FF-FT3 samples had partial overlap along the PC1, and the PC2 could not be distinguished effectively. The results showed that the SF-FT2 and FF-FT3 samples had some similar odor substances. Linear discriminant analysis (LDA) was also performed on odor compounds of different pear samples detected by e-nose, and results are shown in Figure 2C. Overall, the data collected for the different freeze-thaw cycles did not overlap significantly (except FF-FT-1 and FF-FT-2 samples), which indicated that the freeze-thaw cycle had a significant effect on the odorant compounds. However, the formation of these volatiles changing during freezing and thawing needs to be further studied.
Effect of freeze-thaw cycle on the taste of Nanguo pear
An electronic tongue is a multi-sensor system designed to simulate the unique taste perception ability of human beings. It is used to study the five basic tastes (sweet, salty, sour, bitter, and umami) as well as taste sensations including astringency and pungency [Rosa et al., 2017].
Figure 3A shows the radar diagram of sensor response values in Nanguo pears taste analysis using e-tongue. There were no significant differences (p≥0.05) in abundance of aftertaste-A (aftertaste-astringent) and aftertaste-B (aftertaste-bitter) among the 7 samples. There were significant differences (p<0.05) between pears of freeze-thaw groups and fresh pears in sour, bitter, astringent, salty and umami taste. Rapid freezing could produce smaller ice crystals than slow freezing due to the rapid temperature drop [Alabi et al., 2020]; hence, it caused less damage to the structure of fruit cells and tissues, and less cell contents were dissolved, while slow freezing caused greater cell content dissolution, resulting in a large difference in the taste of fast-frozen and slow-frozen Nanguo pears. The results of sour taste analysis showed that, except for the FF-FT1 and SF-FT2 groups, the sourness of pears of the other groups was lower compared to the fresh fruits (Figure 3A). PCA analysis of the signal values of the e-tongue found that PC1 and PC2 represented 47.69% and 32.23% of total variability, respectively (Figure 3B), and the sum of both was 79.92%, indicating that the two principal components were representative. In addition, the distribution of points corresponding to experimental groups in the PCA plot is relatively scattered, which indicates that different freezing methods and the number of freezing and thawing cycles caused differences in the taste of pears. As mentioned above, these differences could be closely related to the ice crystal formation under different freezing modes. At the same time, the freeze-thaw cycle of pears was a complex process, and the pear flavor change was not only due to the ice crystal interaction; therefore, also the deeper reasons need to be further explored.
Effects of freeze-thaw cycles on volatile ester profile of Nanguo pear
Aroma is an important quality feature of fruit. The aroma of fruits was formed by the mixture of many volatile esters, alcohols, aldehydes, ketones and other compounds. The esters are the main volatile compounds that determine the aroma of Nanguo pear [Zhang & Yin, 2023].
Table 2 shows the contents of 24 major volatile esters of Nanguo pear flesh. The total content of esters in fresh pears was 743.86 μg/kg. The sum of esters decreased to 677.74 μg/kg after one freeze-thaw cycle under slow freezing condition. Then, it increased gradually with the subsequent freeze-thaw cycles. After one freeze-thaw cycle under fast freezing condition, the sum of esters of Nanguo pears was 863.36 μg/kg, which was significantly higher than that of fresh fruits and pears of the SF-FT1 group. With the increase of freeze-thaw cycles, the sum of esters continued to increase and then decreased. In flesh, hexyl acetate, ethyl butyrate, ethyl caproate (ethyl hexanoate) and ethyl (E,Z)-2,4-decadienoate were the main esters. The content of ethyl caproate in pear flesh was the highest among esters and ranged from 377.26 to 526.77 μg/kg. This finding is consistent with literature data which reported that ethyl hexanoate was the major volatile compound of Nanguo pears regardless of their ripeness or plant growing locations [Li et al., 2013; Shi et al., 2018]. The odor detection threshold (ODT) value of ethyl caproate is only 1.2 μg/kg [Munafo et al., 2016], indicating that ethyl caproate could be the main compound determining the aroma of Nanguo pears. In the previous studies, it was found that the content of ethyl caproate affected the aroma of pineapple and banana [Dou et al., 2020; Steingass et al., 2014]. As the main detected esters, ethyl hexanoate had the greatest effect on the overall ester content of Nanguo pears, so that the content change trend of total esters after freeze-thaw cycles was consistent with that of ethyl caproate.
Table 2
The content of volatile esters (µg/kg flesh) in fresh Nanguo pears and after three freeze-thaw cycles (FT1, FT2 and FT3, respectively) with freezing at −20°C (SF) and −80°C (FF).
CONCLUSIONS
There were significant differences in the flavor quality of Nanguo pear after three freeze-thaw cycles. The results of analysis performed with the e-nose showed that the freeze-thaw cycle had the greatest effect on nitrogen oxide and hydrocarbons in Nanguo pears. In terms of sour, bitter, astringent, salty and umami taste, there were significant differences between fresh pears and those treated by the freeze-thaw cycles. Due to the repeated melting and recombination of ice crystals, the structure of the fruits was further damaged, resulting in little correlation between fruit flavor and the number of freeze-thaw cycles.