INTRODUCTION
Sweet potato is a dicotyledonous plant with tuberous roots, which belongs to the genus Ipomoea in the family Convolvulaceae and is an important crop species. The root tubers are a rich source of nutrients and bioactive compounds, including starches, proteins, dietary fibers, soluble sugars, carotenoids and anthocyanins, and various minerals such as calcium, iron, phosphorus, and selenium [Tang et al., 2001]. Sweet potatoes rank as the fourth-largest food crop in China, boasting a yearly harvest that surpasses 100 million tons and dominates more than 80% of the world’s output [Mu et al., 2009]. Due to its extensive cultivation and high starch content, it is frequently utilized in the food industry as a crucial raw material for the production of sweet potato starch [Abegunde et al., 2013]. Starch extraction process produces a large amount of sweet potato cell liquid (SPCL) as a discarded by-product, which contains soluble sugars, proteins, and other nutrients [Tang et al., 2001]. This results in significant environmental pollution and the wastage of a valuable nutrient resource. Proteins are one of the main nutrients in SPCL, with a content range of 0.49–2.24 g/100 g [Mu et al., 2014]. Among the sweet potato proteins (SPP), storage proteins (sporamins) are predominant, which account for more than 80% of the total protein [Maeshima et al., 1985], in addition to polyphenol oxidase, β-amylase, and other enzyme proteins [Cheng et al., 2015]. The SPP exhibits a well-balanced amino acid profile and favorable functional properties, such as emulsifying and foaming potential as well as water and oil retention ability [Mu et al., 2017]. Additionally, it exhibits remarkable biological activities including lipid-lowering effects, anticancer potential, and antioxidant activity.
Owing to the high nutritional and application value of SPP, efficient techniques for separation and recovery of proteins from SPCL are important, which can maximize resource utilization and minimize environmental pollution. At present, several techniques have been proposed for the recovery of SPP from SPCL, including isoelectric precipitation [Li et al., 2018], heating precipitation [Mu et al., 2017], ultrafiltration [Zhao et al., 2018], and so on. The isoelectric point protein precipitation is primarily employed. In this method, the pH of SPCL is adjusted to 4.0 through the addition of hydrochloric acid or sulfuric acid, which results in protein precipitation with a yield that ranges from a mere 16.00%, and a corresponding purity level of around 50 g protein/100 g [Arogundade & Mu, 2012]. The yield of isoelectric precipitation could be increased to more than 80% by heating [Mu et al., 2017]. SPCL can also be concentrated using ultrafiltration membranes with a molecular weight cut-off of 10,000 Da, and then SPP is obtained by freeze-drying or adjusting pH of precipitation [Arogundade et al., 2012]. The ultrafiltration method produces relatively high extraction yields (41.30–51.30%) and protein purities (76.00–92.95 g protein/100 g) for SPP [Arogundade & Mu, 2012; Zhao et al., 2018]. However, all the aforementioned techniques are isolation methods used by laboratories to investigate the nature of SPP. At the same time, they are not suitable for the recovery of SPP from SPCL on a larger industrial scale due to the low protein content requiring a large volume of SPCL that needs to be processed.
SPCL is a product of fresh sweet potato processing, carrying a large number of microorganisms. During its storage, the pH gradually decreases to approximately 4.0 due to the spontaneous fermentation, leading to protein precipitation [Li & Mu, 2012]. Manufacturers of sweet potato starch have utilized natural storage fermentation precipitation SPP because it requires no additions and is simple to operate. However, this approach is associated with several drawbacks, including a prolonged protein precipitation period, an unstable process cycle, and suboptimal quality of the recovered SPP.
The present study investigated the feasibility of recovering SPP through artificial inoculation and fermentation. Since the functional properties of proteins play a crucial role in food processing, significantly affecting the texture and sensory properties of food, this study further compared the functional properties of SPPs recovered by isoelectric point precipitation with hydrochloric acid and by fermentation under different conditions. The study results are expected to indicate the possibility for industrial recovery and application of sweet potato protein.
MATERIALS AND METHODS
Materials and reagents
Sweet potato (Ji shu No.25) was provided by Sishui Lifeng Factory in Shandong, China. De Man, Rogosa and Sharpe (MRS) broth medium was purchased from AOBOX Biotechnology Co (Beijing, China). Methanol for high-performance liquid-chromatography (HPLC) was purchased from J&K Sciences, Inc (Beijing, China). Other reagents and solvents were purchased from Sinopharm Chemical Reagent (Shanghai, China). All solutions were prepared with ultrapure water.
The Leuconostoc citreum strain SFZ-T0 was isolated from the spontaneously fermented SPCL in Sishui Lifeng Factory in Shandong and preserved in the collection of the Shandong Jinan Food Fermentation Institute (China).
Preparation of sweet potato cell liquid
SPCL was prepared in accordance with the production process of a sweet potato starch factory as in a previous study by Li et al. [2018]. Fresh sweet potatoes were washed, cut into pieces, mixed with twice their volume of drinking water and pulp. The mixture was then filtered through a 260-mesh filter cloth to remove any residue, after which the pulp was centrifuged at 4,000×g for 5 min. The resulting supernatant served as the experimental SPCL.
Recovery of sweet potato protein by isoelectric point precipitation
The slightly modified acid precipitation method, previously used by Zhao et al. [2019], was applied to recover SPP from SPCL. The pH of SPCL was adjusted to 4.0 using 2 M hydrochloric acid, sulfuric acid, acetic acid, lactic acid, and citric acid, and the mixture was left at room temperature for 2 h. After centrifugation at 6,000×g for 10 min, the precipitate was collected, washed twice with water adjusted to 4.0 by adding a 2 M acid solution, and centrifuged again to remove the supernatant. The precipitate was suspended in deionized water (1:1, w/v) and freeze-dried.
Recovery of sweet potato protein by fermentation
To recover SPP by natural fermentation, the freshly prepared SPCL was incubated at room temperature (25°C) until the pH of the sweet potato juice dropped to 4.0–4.5 and clear stratification of the SPCL occurred. After collecting the precipitate, it was centrifuged at 6,000×g for 10 min to remove the supernatant. The precipitate was suspended in deionized water (1:1, w/v) and freeze-dried.
Inoculation-enhanced fermentation was carried out with the L. citreum SFZ-T0 strain, previously maintained in the laboratory. It underwent two transfers and activations in the MRS medium before being inoculated with a 5% inoculum into the SPCL medium at 30°C for 24 h. The SPCL medium was prepared from sweet potato juice adjusted to a pH of 5.5, heated at 100°C for 10 min, and then centrifuged to remove the precipitate. After autoclaving at 118°C for 20 min, 0.2% yeast extract was added to the clear liquid whose pH was adjusted to 6.0. Subsequently, a 5% inoculate was added into freshly prepared SPCL and incubated at room temperature until the pH of SPCL dropped to 4.0–4.5 and clear stratification of SPCL occurred. After centrifugation at 6,000×g for 10 min, the resulting precipitate was collected, washed twice with water adjusted to pH 4.0, and then subjected to further centrifugation to remove the supernatant. The precipitate was suspended in deionized water (1:1, w/v) and freeze-dried.
After fermentation, the SPCL was heated at different temperatures (40°C, 60°C, 80°C, and 100°C) and centrifuged at 6,000×g for 10 min. The sweet potato protein was obtained by direct heating after the supernatant was removed by centrifugation. In the method of stepwise heating (SH), the protein was first heated to a lower temperature (20°C, 40°C, 60°C, and 80°C), the protein sediment was removed by centrifugation and then heated to a higher temperature (40°C, 60°C, 80°C, and 100°C, respectively), and the supernatant was removed by centrifugation to obtain the sweet potato protein at different segmental temperatures.
Chemical composition analysis
Crude protein content was determined by the Kjeldahl method (N×6.25) [AOAC 1990]. Total sugar content was determined by the phenol-sulfuric acid method [Yue et al., 2022]. Ash content was determined using the scorching constant weight method; the protein products were charred and placed in a muffle oven, where they were ashed at 550°C for 16 h [AOAC 1990].
The lignin content was determined using the method described by Liu et al. [2022]. Respectively weighed 1.0 g of the SPP product was dissolved in 10 mL of a 1% (v/v) acetic acid solution, soaked for 45 min, and filtered. The filtration residue, after washing and drying, was soaked in 4 mL of a mixture of ethanol and ether for a few minutes. After filtration, the precipitate was dried, 3 mL of a 72% (w/v) sulfuric acid solution was added, shaken well and left for about 15 h. Then, the beaker with the mixture was placed in a boiling water bath for a few minutes, and then a 0.5 mL barium chloride solution was added. The obtained precipitate was dissolved in 10 mL of H2SO4 and K2Cr2O7 mixture. After boiling for 15 min, the KI solution was added and titration was performed with an Na2S2O3 solution. The lignin content (LC) was calculated according to Equation (1):
where: K is the concentration of Na2S2O3 (M), a is the volume of Na2S2O3 used during blank titration (mL), b is the volume of Na2S2O3 used during sample solution titration (mL), n is the mass of SPP product (g), and value 48 is equivalent of the titration of 1 mol of lignin (characterized by C11H12O4).Total phenolic content of SPP products was determined using the method described by Velioglu et al. [1988] with slight modifications. SPP products were subjected to ultrasonic extraction with 80% (v/v) methanol for 30 min. The mixture was centrifuged at 2,000×g for 15 min and 200 μL of the supernatant was mixed with 1.5 mL of the Folin–Ciocalteu reagent and 1.5 mL of a sodium carbonate solution (60 g/L). After 90 min at 22°C, the absorbance was measured at 725 nm and results were expressed as gallic acid equivalents (mg GAE/g).
Total flavonoid content of SPP products was determined using the method previously used by Sudewi et al. [2017] with slight modifications. The SPP extract (0.1 g) was first added into 3.0 mL of a 0.1 mM aluminum chloride solution, mixed with vortex oscillation for 3 min, and left for 30 min at room temperature. The absorbance of the mixed solution was measured at the wavelength of 415 nm, and the result was expressed in rutin equivalent (mg RE/g).
The sugar profile of the supernatant after SPCL fermentation was determined using high-performance liquid chromatography (HPLC). The supernatant samples were appropriately diluted, filtered through a 0.45 μm membrane, and then analyzed on a U3000 HPLC system (ThermoFisher Scientific, Waltham, MA, USA). The sugars were separated on a Waters Sugar-Pak column (6.5×300 mm, particle diameter 10 μm; Waters Company, Milford, MA, USA) using 0.01 M ethylenediaminetetraacetic acid calcium disodium salt hydrate as a mobile phase with a flow rate of 0.6 mL/min. Injection volume was 10 μL, and column temperature was 80°C. A differential refractive index detector (RI) was used for detection. The identification of sugars was performed by comparing their peak retention times with those of sugar standards, and the quantification was based on the peak areas.
Sweet potato protein functional properties analysis
Solubility
The solubility of SPP was determined according to the method described by Mu et al. [2009] with slight modifications. An aliquot of 1.0 g of the SPP product was added to 100 mL of distilled water and stirred for 1 h at room temperature. After centrifugation at 4,500×g for 10 min, the supernatant was collected to determine the protein content by the Kjeldahl method [AOAC, 1990]. The solubility (%) was calculated according to Equation (2):
where: m1 (g) and m0 (g) represent the protein content of the supernatant and the SPP product, respectively.Emulsifying properties
The emulsifying activity index (EAI) and emulsion stability index (ESI) of SPP products were determined according to the method described by Cui et al. [2021] with slight modifications. A 1% (w/v) aqueous solution of the SPP sample was prepared. An aliquot of 30 mL of the aqueous solution was mixed with 10 mL of peanut oil and homogenized for 90 s at 10,000 rpm to form an emulsion. The resulting emulsions were left undisturbed for 0 and 20 min before taking a sample of 20 μL, which was then mixed with 5 mL of a 0.1% sodium dodecyl sulfate solution. The absorbance was measured at 500 nm using a spectrophotometer (SH-6600, Jiangsu Sheng Aohua Environmental Protection Technology Co. Ltd, Shanghai, China). The EAI (m2/g) and ESI (min) were calculated according to Equations (3) and (4), respectively:
where: D is the dilution factor (250), l is the length of the optical path (1cm), φ is the oil volume fraction (0.25), C is the amount of protein per unit volume before emulsification (g/mL), A0 is the absorbance value at 0 min of standing, A20 is the absorbance value at 20 min of standing, and t is the standing time (20 min).Foaming properties
The foaming capacity (FC) and foaming stability (FS) of SPP products were determined according to the method described by Kim et al. [2021] with slight modifications. A 1% (w/v) aqueous solution of the SPP product (75 mL) was vigorously stirred using a disperser at 10,000 rpm for 2 min to ensure complete protein foam formation. The initial volume of the solution before and after stirring was recorded, followed by another measurement after storage for 30 min. The FC and FS were calculated according to Equations (5) and (6), respectively:
where: V1 and V2 are the volumes (mL) of the dispersion before and after stirring, respectively, V3 is the volume (mL) of the protein solution after storage for 30 min at room temperature after stirring.Sweet potato protein digestibility analysis
The SPP product (2 g) was transferred into a 50 mL centrifuge tube; then, 30 mL of a pepsin solution (enzyme activity of 2,000 U/g) was added, and the mixture was incubated at a temperature of 37°C for 6 h. Subsequently, 10 mL of a trichloroacetic acid solution with a concentration of 10% (wv) was added to precipitate any undegraded protein present in the mixture, which was then subjected to centrifugation at 6,000×g for 10 min before collecting the resulting precipitate. The collected precipitate was washed twice using water acidified to pH 4.0 and freeze-dried thereafter. The digestibility was calculated according to Equation (7) [Chen, 2023]:
where: m1 (g) and m0 (g) are the weights of precipitated protein and the SPP product, respectively.Statistical analysis
All experiments were conducted in triplicate, and the results were expressed as mean and standard deviation. Statistical analysis was performed using one-way analysis of variance (ANOVA) with SPSS software (IBM, Shanghai, China) at a significance level of p<0.05. Graphical representation was generated using Origin 2018 (Origin Lab, Northampton, MA, USA).
RESULTS AND DISCUSSION
Separation of sweet potato proteins by precipitation with acids
Currently, the isoelectric point precipitation method stood as the most commonly employed technique for SPP recovery from SPCL in research [Arogundade & Mu, 2012; Li et al., 2018]. The pH value of SPCL is usually adjusted to about 4.0 with hydrochloric acid to precipitate SPP and realize protein recovery. In this study, several inorganic and organic acids commonly utilized in the industry (for example, citric acid and acetic acid are widely used in food production as sour agents) were selected to adjust the pH of SPCL for SPP precipitation. The results showed that organic acids, such as lactic acid and citric acid, could also be used for SPP precipitation, with marginally improved rates of SPP recovery and protein purity compared to the inorganic acids (Table 1). The protein content of SPCL was 0.35 g/100 g. It can be calculated that the production of 1,000 kg SPP product required a significant amount of hydrochloric acid (375 L), and even more of lactic and citric acids (750 L). Therefore, there was a substantial demand for acids for SPP separation by pH adjustment, which not only incurred high raw material costs but also generated new sources of pollution without any commercial application potential.
Table 1
Extraction yield and purity of sweet potato protein (SPP) products from sweet potato cell liquid (SPCL) obtained by isoelectric point precipitation with different acids.
Separation of sweet potato protein by microbial fermentation regulating pH and precipitation
During SPCL storage, microorganisms (primarily lactic acid bacteria) may reproduce and cause the SPCL to become acidic, eventually reaching the pH required for protein precipitation [Li & Mu, 2012; Pagana et al., 2014]. However, the composition of natural fermentation microorganisms fluctuates greatly (there are many species in the natural fermentation system, so the growth of species is uncontrollable), leading to prolonged and unstable fermentation times, low levels of lactic acid bacteria, and a slower pH decrease [Capozzi et al. 2017]. These factors can contribute to SPCL spoilage and protein precipitation failure. In view of this situation, in the early stage, we isolated and screened the SFZ-T0 strain from the natural fermentation sedimentation tank of SPCL, which can rapidly propagate Leuconostoc citreum in SPCL (data not shown). Afterwards, we investigated the possibility of precipitating and separating SPP from SPCL through fermentation with the inoculation strain SFZ-T0.
Firstly, a comparison was made between the pH values of SPCL fermented through natural fermentation and those inoculated with strain SFZ-T0. The results shown in Figure 1 indicate that while the pH of naturally fermented SPCL remained unchanged for 4–6 h, it began to decrease after this period and eventually stabilized after 24 h. In contrast, the SPCL inoculated with strain SFZ-T0 exhibited an immediate decline in pH post-inoculation which dropped to about 4.0 within 6–8 h. Meanwhile, the fermentable sugars, such as sucrose, glucose and fructose, in SPCL were rapidly consumed (Table 2), indicating that strain SFZ-T0 utilized these sugars for acid production, resulting in a rapid decrease of pH in the feed solution to achieve the purpose of protein precipitation. Instead of increasing, the yield and purity of SPP showed a slight decrease with prolonged fermentation time (Table 3), which may be attributed to the bacterial consumption of protein. The optimal extraction yield and purity of SPP were achieved at a fermentation time of 6–8 h.
Figure 1
Changes in pH during inoculation-enhanced fermentation and natural fermentation of sweet potato cell liquid.
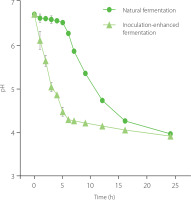
Table 2
Soluble sugar profile of sweet potato cell liquid unfermented, fermented naturally and fermented with inoculation (g/L).
Table 3
Extraction yield and purity of sweet potato protein (SPP) products obtained by inoculation-enhanced fermentation of sweet potato cell liquid (SPCL) at different times.
Inoculation-enhanced fermentation could rapidly precipitate SPP, but it required strain expansion for each batch of inoculation. If each batch of fermentation was inoculated with seed liquid, the cost and difficulty of industrialization would increase. Therefore, this study explored the feasibility of continuous transfer of fermentation broth instead of batch inoculation. As shown in Figure 2, a total of 10 batches with 9 transfer experiments were conducted at a transfer rate of 20%. The results showed that with an increase in the number of transfers, the rate of pH reduction of the SPCL feed increased rather than decreased. The time taken to reach the isoelectric point shortened from approximately 6 h in the first batch to about 5 h in the ninth batch.
Therefore, inoculating strain SFZ-T0 enhanced fermentation, and continuous transfer for SPP recovery from SPCL was a simple, cost-effective method with a commercial application value.
Effect of heat treatment on sweet potato protein recovery by microbial fermentation
SPP could be effortlessly and efficiently recovered from SPCL through inoculation-enhanced fermentation, resulting in a higher extraction yield and purity compared to the isoelectric precipitation with hydrochloric acid (extraction yield of 52.70% and 47.58%, respectively, purity of 61.43 and 59.43 g protein/100 g, respectively) (Table 1 and 4). Although the extraction yield of SPP was slightly higher than that reported in the literature for medium isoelectric point precipitated proteins [Arogundade & Mu, 2012], it still remained below 60%, indicating a significant amount of unprecipitated proteins. Heat treatment is a commonly employed technique for improving protein separation and extraction. Mu et al. [2017] achieved an extraction yield of over 80% for SPP by utilizing a combination of isoelectric point precipitation and heat treatment. Therefore, we investigated the effect of heat treatment on the yield of inoculation-enhanced protein fermentation.
The fermentation broth was heated to different indicated temperatures and then centrifuged to separate the proteins. The results showed that thermal processing significantly enhanced the protein extraction yield (Table 4). When the fermented SPCL was heated from room temperature to 40°C, the yield of SPP extraction increased by around 20% compared to that without heating. Upon reaching a temperature of 100°C, the SPP extraction yield peaked to 95.34%, which was accompanied by an increase in purity. In addition, we conducted a comparison of the yield and purity of SPP extracted through stepwise heating (Table 5) and found out that as the heating temperature increased, the purity of SPP products also increased. The purity of SPP isolated at 80/100°C reached 95.19 g protein/100 g.
Table 4
Extraction yield and purity of sweet potato protein (SPP) products obtained by direct heat treatment of sweet potato cell liquid (SPCL) after inoculation-enhanced fermentation.
SPP product | Yield (%) | Purity (g protein/100 g) |
---|---|---|
F-SPP | 56.78±0.34e | 66.23±0.38d |
SPP40 | 76.97±0.28d | 66.36±0.32d |
SPP60 | 85.42±0.37c | 67.15±0.80c |
SPP80 | 91.45±0.25b | 68.62±0.98b |
SPP100 | 95.34±0.31a | 70.12±1.63a |
[i] F-SPP, SPP product obtained by inoculation-enhanced fermentation; SPP40, SPP60, SPP80 and SPP100, SPP products obtained by direct heating SPCL to 40°C, 60°C, 80°C and 100°C after inoculation-enhanced fermentation, respectively. Different letter superscripts in the same column indicate significant differences (p<0.05).
Table 5
Extraction yield and purity of sweet potato protein (SPP) products obtained by stepwise heat treatment after inoculation-enhanced fermentation.
SPP | Yield (%) | Purity (g protein/100 g) |
---|---|---|
F-SPP | 56.78±0.34a | 66.23±0.38d |
SH-SPP20/40 | 20.19±0.31b | 67.41±0.32d |
SH-SPP40/60 | 8.45±0.32c | 75.43±0.80c |
SH-SPP60/80 | 6.03±0.31d | 86.49±0.98b |
SH-SPP80/100 | 3.89±0.28e | 95.19±1.63a |
[i] F-SPP, SPP product obtained by inoculation-enhanced fermentation; SH-SPP20/40, SH-SPP40/60, SH-SPP60/80 and SH-SPP80/100, SPP products obtained by stepwise heating SPCL to 20 and 40°C, 40 and 60°C, 60 and 80°C, and 80 and 100°C after inoculation-enhanced fermentation, respectively. Different letter superscripts in the same column indicate significant differences (p<0.05).
Chemical composition of sweet potato proteins
As indicated in Table 6, SPP products contained carbohydrates, which had been further analyzed as starch, in addition to protein. Furthermore, SPP products also comprised a small Table 6. Chemical composition of sweet potato protein (SPP) products. quantity of ash, lignin, and bioactive compounds such as phenolics. The chemical composition of SPP products was basically the same compared with the report in the related literature, although the proportions between compound contents were slightly different [Arogundade & Mu, 2012]. First of all, the protein content of SPP products after fermentation and heating in our study was significantly higher than that of SPP products extracted by isoelectric point precipitation and ultrafiltration reported in literature [Arogundade et al., 2012]. Due to the low protein content of sweet potato protein recovered by isoelectric point precipitation, the proportion of non-protein compounds in SPP isolated by this method (S-SPP) was relatively high (Table 6). This observation is in accordance with the literature data [Arogundade & Mu, 2012]; the low content of medium electric point precipitated protein correlated with high total phenolic content (3.76 mg GAE/g) and total flavonoid content (3.48 mg CE/g). The purity of SPP isolated through heating increased with temperature elevation while the proportion of non-protein components decreased significantly. Although the purity of the directly heated SPP product was higher, it was still inferior to the stepwise heating, which was caused by the centrifugal removal of the low-purity protein when heated at low temperature, but the content of non-protein compounds of the directly heated protein was higher.
Table 6
Chemical composition of sweet potato protein (SPP) products.
[i] S-SPP, SPP product obtained by acid precipitation; F-SPP, SPP product obtained by inoculation-enhanced fermentation; SPP40, SPP60, SPP80 and SPP100, SPP products obtained by direct heating sweet potato cell liquid (SPCL) to 40°C, 60°C, 80°C and 100°C after inoculation-enhanced fermentation, respectively; SH-SPP20/40, SH-SPP40/60, SH-SPP60/80 and SH-SPP80/100, SPP products obtained by stepwise heating SPCL to 20 and 40°C, 40 and 60°C, 60 and 80°C, and 80 and 100°C respectively; GAE, gallic acid equivalent; RE, rutin equivalent. Different letter superscripts in the same column, separately for SPP and SH-SPP samples, indicate significant differences (p<0.05). S-SPP and F-SPP were statistically analyzed with direct heating and stepwise heating, respectively.
Functional properties of sweet potato proteins
Solubility
Solubility is a crucial indicator for evaluating the functional properties of plant proteins, and favorable solubility is essential for the functional and nutritional value of the product [Yang et al., 2018]. Table 7 shows the solubility values of SPP extracted using different methods. The solubility of SPP isolated by isoelectric point precipitation and inoculation-enhanced fermentation (S-SPP and F-SPP, respectively) was found to be 72.35% and 85.46%, respectively. The main reason for this difference was that the protein content of F-SPP was higher, and more protein was dissolved under the same conditions. The heat treatment significantly (p<0.05) decreased the solubility of SPP. It was due to the fact that heat treatment could destroy the structure of the protein, which makes it difficult to maintain the original solubility. However, protein extracted by heating below 60°C still exhibited a solubility above 50%, which provided the possibility of SPP functional application.
Table 7
Solubility of sweet potato protein (SPP) products.
[i] S-SPP, SPP product obtained by acid precipitation; F-SPP, SPP product obtained by inoculation-enhanced fermentation; SPP40, SPP60, SPP80 and SPP100, SPP products obtained by direct heating sweet potato cell liquid (SPCL) to 40°C, 60°C, 80°C and 100°C after inoculation-enhanced fermentation, respectively; SH-SPP20/40, SH-SPP40/60, SH-SPP60/80 and SH-SPP80/100, SPP products obtained by stepwise heating SPCL to 20 and 40°C, 40 and 60°C, 60 and 80°C, and 80 and 100°C respectively. Different letter superscripts in the same column, separately for SPP and SH-SPP samples, indicate significant differences (p<0.05). S-SPP and F-SPP were statistically analyzed with direct heating and stepwise heating, respectively.
Emulsifying properties
The formation of emulsions required emulsifiers to mix two immiscible liquids, and proteins had long been used as effective emulsifying agents [McClements, 2004]. The emulsifying activity index (EAI) and the emulsion stability index (ESI) were typically used to assess the protein’s ability to form stable emulsions [Kumar et al., 2014]. The results of EAI and ESI analyzed for SPP extracted by different extraction methods are shown in Table 8. The EAI of F-SPP (28.30 m2/g) was significantly (p<0.05) higher than that of SPP extracted after stepwise heat treatment (2.10–21.34 m2/g), which was significantly related to the solubility of SPP. It was also better than that of hemp seed protein (8.7 m2/g) [Yao et al., 2023], potato protein (22.80 m2/g) [Hussain et al., 2021] and soybean protein (26.43 m2/g) [Ding, 2021]; most of these different plant proteins were isolated by acid precipitation. The ESI trends of different SPP products differed significantly from their EAI. SPP separated below 60°C showed good emulsion stability. The ESI of SH-SPP40/60 was better than that of F-SSP, and the ESI of SPP separated above 80°C decreased rapidly. Appropriate heat treatment could gradually expose the hydrophobic groups in protein molecules, thereby enhancing the hydrophobic interaction between interfacial proteins and promoting emulsion stability. However, high temperature (80–100°C) may lead to complete denaturation of the sweet potato protein, resulting in protein precipitation and reducing the degree of protein adsorption at the water-oil interface, thereby reducing the stability of the emulsion.
Foaming properties
Foam could alter both the texture and flavor of food, with proteins exhibiting favorable foaming properties. The foaming capacity (FC) and foam stability (FS) are two important parameters of protein foaming properties. The foaming properties of sweet potato proteins obtained by different extraction methods are shown in Table 8. The F-SPP had the FC of 38.49%, which was higher than that of the SPP isolated via isoelectric point precipitation by Mu et al. [2017] (FC 30–35%), and other proteins, such as potato protein (FC 20%) [Hussain et al., 2021], peanut protein (FC 26.47%) [He et al., 2023] and mung bean protein (FC 26.10%) [Du et al., 2018]; most of these different plant proteins were isolated by acid precipitation. The results of various stability tests performed for SPP foam were consistent with its foaming ability, which was affected by the reduced solubility caused by heating and subsequently influenced its foam stability [Mir et al., 2021]. F-SPP exhibited good foaming properties in the light of literature data for plant-derived protein isolates [Soria-Hernández et al., 2015].
Table 8
Emulsifying and foaming properties, and digestibility of sweet potato protein (SPP) products.
[i] SPP, SPP product obtained by acid precipitation; F-SPP, SPP product obtained by inoculation-enhanced fermentation; SH-SPP20/40, SH-SPP40/60, SH-SPP60/80 and SH-SPP80/100, SPP products obtained by stepwise heating SPCL to 20 and 40°C, 40 and 60°C, 60 and 80°C, and 80 and 100°C respectively. Different letter superscripts in the same column indicate significant differences (p<0.05).
Digestibility of sweet potato proteins
Digestibility is a crucial nutritional characteristic of the food protein. The higher the digestibility, the better the protein utilization rate and the easier its digestion and absorption. The digestibility of SPP products obtained by different extraction methods are shown in Table 8, indicating that the heat treatment significantly enhanced SPP digestibility. Specifically, when heated to 60°C, the digestibility of SPP exceeded 87%, which was 7.32% higher than that of the F-SPP samples. Moreover, heating at 100°C further increased the digestibility of SH-SPP80/100 to reach up to 90%. This is due to the fact that the high temperature treatment destroys the structure of the protein itself and significantly improves SPP digestibility [Sun et al., 2012], although on the other hand, the functional properties of SPP extracted by heat denaturation may be lost. SPP had been heat-treated after being purified (to a purity of 95 g protein/100 g) by Sun et al. [2014]. As the temperature increased, its digestibility increased from 52.8% to 99.6% (at 110°C), while that of the sample heated at 100°C was approximately 80%. Although SPP isolated by heating at higher temperatures (80–100°C) could have worse functional properties, it boasted high protein content and excellent digestibility, rendering it an ideal source of high-quality protein.
CONCLUSIONS
In this study, SPP was recovered from SPCL by artificial inoculation-enhanced fermentation acidification and heating, and the functional properties of the prepared SPP were compared. The results showed that within 6 h of inoculation-enhanced fermentation, the pH level of SPCL could be reduced to less than 4.0, leading to the successful settling of SPP. The extraction yield and purity could be achieved at approximately 55% and 65 g protein/100 g, respectively. Heat treatment at low temperatures (40–60°C) increased the protein extraction yield to 75–85%, while SPP maintained high emulsifying and foaming properties. High-quality SPP products with a protein content ranging from 86 to 95 g/100 g and a digestibility exceeding 90% could be obtained by stepwise heat treatment at high temperatures (60/80–80/100°C).
The present study described a cost-effective and straight-forward approach for promoting the industrial recovery of SPP from SPCL through inoculation-enhanced fermentation and continuous transfer. Additionally, the application of assisted heat treatment could produce high-quality SPP products with diverse functional properties, which held a promising application prospect in the food industry.