ABBREVIATIONS
O/W, oil-in-water; %EE, encapsulation efficiency; NB, natural beads; BB, beetroot beads; DWB, dressing without beads; DNB, dressing with natural beads; DBB, dressing with beetroot beads; COD, commercial dressing; V24h, viability after 24 h; LAB, lactic acid bacteria; cfu, colony forming unit.
INTRODUCTION
Probiotics such as Lactiplantibacillus plantarum are part of human intestinal microbes. They play a very important role in food fermentation processes [Nordström et al., 2021]. In addition, they are live microorganisms which can confer health benefits by improving the host’s intestinal microbial balance when administered in adequate amounts [Baek & Lee, 2009]. Likewise, probiotics are being commercialized in many different forms, including supplements or fermented foods from animal or vegetal origin (dairy and non-dairy products, respectively). L. plantarum 299v is the most documented and popular L. plantarum strain in the world [Nordström et al., 2021]. The consumption of probiotic foods is an actual demand and these products account for up to 70% of the total functional food market [Rajam & Subramanian, 2022]. On the other hand, prebiotics are defined as ingredients that promote the growth or metabolic activity of the beneficial bacteria; when probiotics and prebiotics are in combination, they are known as “synbiotics” and are designed to improve the survival of the microorganisms and their colonization [Vijaya Kumar et al., 2015]. The trend of incorporating natural sources of bioactive compounds to obtain healthier food products has led researchers to investigate their prebiotic potential [Lazar et al., 2022].
The beetroot (Beta vulgaris L.) is rich in bioactive compounds and confers health benefits due to its antioxidant and antiinflammatory effects, among other features [Chhikara et al., 2019; Mirmiran et al., 2020]. It is applied as a functional ingredient due to its content of betalains, flavonoids, phenolic acids, and saponins [Otalora et al., 2020; Platosz et al., 2020; Spórna-Kucab et al., 2022]. Moreover, its benefits on the gut microbiota, due to the content of oligo- and polysaccharides, have also been demonstrated and therefore it has received special attention as a potential prebiotic [Alexandrino de Oliveira et al., 2021].
The encapsulation of probiotic cells serves to protect them from hostile environments; this is how they can reach the intestine without being damaged. On the other hand, the probiotic cell is immobilized and could be added to a product in order to extend the cell viability, along with its shelf life [Vijaya et al., 2015]. Dressings are oil-in-water (O/W) emulsions which are widely consumed by the population; for instance, a variety of products such as mayonnaise or dressings are available in the market. The tendency to consume foods based on natural ingredients compels producers to incorporate ingredients from vegetal origin into dressings that could improve their functional properties [Castillo et al., 2021]. The quality of the final product based on O/W emulsions is governed by diverse rheological characteristics that can be affected during the incorporation of new ingredients [Ma & Boye, 2013]. Due to the reasons stated above, the aim of the present research was to study the viability of L. plantarum co-encapsulated with beetroot extract loaded into alginate beads, and the effect of capsules on rheological properties when incorporated as an ingredient in a model food (O/W emulsion). This information may provide valuable data for the development of new, healthier products.
MATERIALS AND METHODS
Microbial strain and culture conditions
A bacterial strain of Lactiplantibacillus plantarum 299v (L. plantarum Orla-Jensen 1919 Bergey et al. 1923 strain 17-5) was acquired from the American type culture collection (ATCC; Manassas, VA, USA) and donated by the Laboratory of Cell Biology of the Autonomous University of Nuevo León (San Nicolás de los Garza, Mexico). It was kept on De Man Rogosa Sharpe (MRS) broth (Difco, Becton & Dickinson, Sparks, MD, USA) in refrigeration until use. In order to obtain fresh cultures, an aliquot of 100 μL was taken from the refrigerated culture and placed in tubes containing 10 mL of MRS medium; they were incubated at 37°C for 24 h for further analyses. For the encapsulation, the cells were recovered by centrifugation (Hermle Labnet Z326, Labnet International, Inc., Wehingen, Germany) at 590×g for 20 min at 25°C and washed twice with 10 mL of 8.5 g/L of a saline solution. The cells were then suspended in 1 mL of the saline solution and used for the respective encapsulation method. A plate count method in MRS was used to determine the final count of cells in the suspension [Mahmoud et al., 2020].
Preparation of beetroot material
The beetroots (Beta vulgaris L.) were purchased from retail markets in the metropolitan area of Monterrey, NL, Mexico. They were washed and disinfected with mycrodin® (Tavistock Group, Mexico City, Mexico) for 15 min. After that, the peel was separated and discarded. Then, the beetroots were chopped into small pieces using a knife (squares of 0.5 cm) and placed in a tunnel drier (model LO2001, Procomm, Mexico City, Mexico) at 45°C for 24 h. The dried material was ground in a mill (model 4 Wiley mill, Thomas Scientific, Swedesboro, NJ, USA) with a mesh of 1 mm and stored in a dry, sterile container protected from light [Castillo et al., 2021]. This material was used later for the beetroot extract preparation.
Preparation of the beetroot extract
An aqueous extract of dried beetroot powder was prepared according to the procedure described by Sánchez et al. [2016], with minor modifications. Briefly, the dried material was soaked in sterile distilled water (20 g/L) and left at room temperature (25°C) for 48 h in a sterile screw-capped glass flask. After that, the aqueous material was recovered by filtration (Whatman No.1) and placed in a sterile glass container at 4°C until use. Finally, the extract was incorporated into the capsules according to the method mentioned below.
Preparation of capsules and their characterization
Two methods, including emulsification and extrusion, were used for the encapsulation of L. plantarum with alginate (natural beads, NB) and co-encapsulation of L. plantarum and beetroot extract with alginate (beetroot beads, BB). The particle size and morphology of the capsules obtained by both methods were determined. The encapsulation efficiency (%EE) was determined only for the method that gave the best results in terms of particle size and morphology of capsules.
Encapsulation by emulsification
The emulsification method was carried out according to the procedure described by Motalebi Moghanjougi et al. [2021], with minor modifications. First, a sodium alginate solution (10 g/L) was prepared and autoclaved (121°C/15 min). After that, 1 mL of the harvested cells of L. plantarum in saline solution was mixed with 99 mL of the alginate solution. The mixture (20 mL) was then added dropwise with a sterile syringe with needles of different calibers (G22×32 mm, G27×13 mm, G31×6 mm), to a previously sterilized solution containing 100 mL of vegetable oil (canola) with 0.1% (w/v) Tween 80 (Sigma-Aldrich, Saint Louis, MO, USA); then, it was magnetically stirred for 5 min. Subsequently, 100 mL of cooled 0.15 M CaCl2 were added slowly to the mixture to break the emulsion. The mixture was left at room temperature for 30 min and the capsules were formed. The oil layer was removed, and the probiotic beads were recovered by filtration (Whatman No.1). The beads were washed twice with 0.15 M CaCl2 and stored at 4°C for the analyses. For the incorporation of the beetroot extract to the bead, the same procedure mentioned above was used, but part of the sterile alginate solution (10 mL) was replaced by the same volume of the plant extract.
Encapsulation by extrusion
The encapsulation of L. plantarum through the extrusion technique was performed according to the method reported by Mahmoud et al. [2020] and Krasaekoopt & Watcharapoka [2014], with some modifications. Briefly, 1 mL of the harvested probiotic culture of L. plantarum was added to 99 mL of alginate solution (10 g/L) previously prepared and autoclaved. The suspension was extruded dropwise with a syringe through needles of different calibers (G22×32mm, G27×13mm, G31×6mm) in a sterile 0.15 M CaCl2 with gentle magnetic stirring. The encapsulated cells were then washed with 0.15 M CaCl2 and stored in a sealed bag at 4°C in sterile peptone water. For the incorporation of the beetroot extract to the beads, the plant extract (10 mL) was added to the alginate solution (89 mL). After adding 1 mL of the L. plantarum suspension, the same procedures of extrusion and capsule washing were used as described above.
Analysis of particle size and morphology of capsules
The average diameter of particles and the morphology of the capsules were evaluated by optical microscopy using a Leica EZ4HD digital stereo microscope with a high-definition CMOS camera (Leica, Wetzlar, Germany) according to the method reported by Krasaekoopt & Watcharapoka [2014] and Holkem et al. [2016]. Magnification of 10× was used. The diameters of 100 randomly selected capsules were measured using the Image-Pro Plus 2D analysis software (Media Cybernetics, Rockville, MD, USA) with the caliper tool.
Encapsulation efficiency determination
Once the capsules were obtained using the protocol that produced the best results in particle size and morphology, the encapsulation efficiency as a function of percentage of entrapped cells was determined according to Krasaekoopt & Watcharapoka [2014]. Briefly, 1 g of fresh beads was blended in a stomacher for 1 min with 99 mL of sterile peptone solution (1 g/L) and then allowed to stand for 10 min to dissolve. Appropriate serial dilutions were made and then a plate count method was deployed onto MRS broth (Difco, Becton & Dickinson). The same procedure was carried out after 24 h of the encapsulation for monitoring the viability of L. plantarum in order to discard any antimicrobial activity.
The encapsulation efficiency (%EE) was calculated according to Equation (1), as suggested by Pupa et al. [2021].
where: N0 is the initial population of L. plantarum before encapsulation, and N is the population of encapsulated L. plantarum.The viability of bacteria after 24 h (%V24) was calculated from Equation (2):
where: N is the initial population of encapsulated L. plantarum, and N24 is the remaining encapsulated population after 24 h.Determination of L. plantarum viability under simulated gastrointestinal conditions
In order to determine the viability of L. plantarum under the gastrointestinal conditions, the method mentioned by Krasaekoopt & Watcharapoka [2014] and Chávarri et al. [2010] was used with minor modifications. One gram of freshly prepared beads was incubated in a tube containing 10 mL of simulated gastric juice (0.2% NaCl, 0.08 M of HCl, without pepsin and adjusted to pH 1.5 with HCl). This mixture was incubated at 37°C for 90 min; afterwards, the beads were recovered by filtration and resuspended in 9 mL of sterile simulated intestinal juice containing saline (0.5%) with pancreatin (0.1%) and bile salts (0.5%) at pH 8. This mixture was incubated at 37°C for 120 min. At the end of the process, the capsules with probiotics were recovered to determine their viability by means of a plate count (log cfu/g) as described above.
Determination of rheological properties and physical stability of model food with the capsules
A dressing (O/W emulsion) was used as model food. After addition of the capsules, the rheological properties, physical stability of model food, and the viability of encapsulated probiotics were determined throughout 28 days.
Preparation of the O/W emulsion food model
The ingredients used to formulate the O/W emulsion are presented in Table 1. The procedure performed according to Castillo et al. [2021] is described as follows: first, the water and egg yolk were placed in a bowl and mixed at 500 rpm for 5 min (T50 Digital Ultra Turrax, IKA, Wilmington, NC, USA). After that, vinegar, salt, sugar, and xanthan gum were added and mixed for 15 more min. Finally, the oil was added manually in a continuous manner until it was fully incorporated into the mix at 3,000 rpm for 2 min, and at 5,000 rpm for 3 more min. Once the emulsion was finished, the beads were added according to the formulations reported in Table 1. The first formulation was the dressing without the addition of beads (DWB), the second one was the dressing with the addition of natural beads (DNB), and the third one was the dressing with the addition of beetroot beads (DBB). The formulated dressings were packed in a sealed bag and stored at 4°C.
Table 1
Composition (g/100 g) of oil-in-water emulsion dressings without beads (DWB), and with natural beads (DNB) obtained by encapsulation of Lactiplantibacillus plantarum with alginate and with beetroot beads (DBB) obtained by co-encapsulation of L. plantarum and beetroot extract with alginate.
Analysis of flow properties of the O/W emulsion food model
The flow curves of the O/W emulsions (DWB, DNB and DBB) were obtained for the shear rate in range from 1 to 100 1/s using a rotational test with a RheolabQC rheometer (Anton Paar, Mexico City, Mexico) and a CC27 geometry at a temperature of 25°C [Castillo et al., 2021]. Consistency indexes (k, Pa×sn) and flow behavior indexes (n) were obtained according to the power law model described in Equation (3) [Sakin-Yilmazer et al., 2014].
The commercial dressing was used as a point of comparison. Analyses were carried out for fresh dressings and dressings stored for 28 days.
Droplet size and coalescence rate determination
The particle size of fresh emulsions and those stored for 14 and 28 days were monitored with the Malvern Mastersizer 3000 equipment (Malvern Instruments, Ltd, Worcestershire, UK) using the Hydro LV unit with water as a dispersant. Angular scattering intensity data from the analyzer were used to calculate the size of the particles that create the scattering pattern using Mie’s theory of light scattering [Bautista Villarreal et al., 2018]. The software Malvern Mastersizer v3.63 (Worcestershire, UK) was used to calculate the Sauter mean diameter (d3,2). The optical properties of the emulsions were defined as follows: the refractive index was 1.460, while the absorption index was 0.100. The coalescence rate (Kc, 1/s) was obtained based on the first order kinetics and the Equation (4).
where: Nt is the concentration in the number of drops at a time = (t), N0 is the concentration in the number of the newly formed drops (time = 0), and Kc is the rate constant, which is related to the probability that the interfacial film will break at a certain time (t). The volume of the emulsion droplets remains constant when there is no oil release in the emulsion [Castillo et al., 2021].Determination of L. plantarum viability in the food model under storage conditions
In order to monitor the viability of encapsulated L. plantarum over time in the food model, a plate count technique was used. Briefly, after preparation of the dressing, a portion of 10 g containing 1 g of respective beads was packed in a sealed bag and stored at 4°C. Each sample was processed in time intervals (on day 0, 2, 4, 8, 12, 18, 22 and 28), by serial dilutions and plating. Results were expressed as log cfu/g.
RESULTS AND DISCUSSION
Beetroot was selected as vegetal material to be included in the encapsulation of L. plantarum since it has been implicated in different health benefits and recognized as an important source of antioxidants [Chhikara et al., 2019]. It has also been reported as functional food [Chhikara et al., 2019; Platosz et al., 2020]. Beetroot has become a very popular food and actually is one of the top ten planted vegetables associated with superior health benefits [Borjan et al., 2022]. On the other hand, some investigations have suggested the possibility of beetroot juice encapsulation to preserve its functional characteristics [Tumbas Šaponjac et al., 2020]. For this reason, in this research, beetroot aqueous extract was obtained and co-encapsulated with L. plantarum.
Characterization of capsules
Size and morphology
Considering that particle size can influence the aroma, texture, and appearance of the food, it may be deemed a very important physical parameter [Holkem et al., 2016]. In our study, the alginate beads loaded with L. plantarum and with L. plantarum and beetroot aqueous extract varied in size and morphology depending on the protocol of capsule preparation used. However, the particle size was independent of the addition of beetroot extract (data not shown). The extrusion technique derived in spherical alginate beads (Figure 1) with an average particle diameter between 2,260 to 978 μm (for capsules with L. plantarum and beetroot extract), depending on the needle caliber used (Table 2). In general, the beads obtained by this protocol presented a homogeneous size for each needle caliber. On the other hand, beads obtained by the emulsion technique had an irregular morphology (Figure 1) and differed greatly in diameters. The average particle diameter of these beads ranged from 600 to 2,850 μm (Table 2). The smaller beads in size were obtained by the emulsification and needle calibers of G31×6 mm, G27×13 mm compared to analogous beads obtained using the extrusion technique. These results are in agreement with Capela et al. [2007], who reported a wide size distribution of alginate capsules loaded with Lactobacillus acidophilus, L. casei and L. rhamnosus when using the emulsion technique with bead sizes ranging from 200 to 1,000 μm. Also, Li et al. [2009] and Lopes et al. [2017], reported more uniform alginate beads loaded with probiotics obtained by the extrusion technique with diameters ranging from ~900 to 1,400 μm, which is consistent with our study results. Pupa et al. [2021] reported varied alginate-chitosan bead shapes and sizes depending on the preparation technique used, with mean diameters of ~1,500 μm for those obtained by extrusion, and ~500 μm for those obtained by emulsion technique, while Mahmoud et al. [2020] obtained alginate microcapsules loaded with L. plantarum and biopolymers by the extrusion technique with sizes ranging from ~501 to 1,200 μm. According to Holkem et al. [2016], particle sizes below 100 μm are desirable to avoid the negative impact on the sensory characteristics; however, some marketing strategies regarding flavor may help in the acceptance of the product. Nevertheless, the optimum size of the microcapsules could be debatable as it varies according to their applications. Actually, encapsulating lactic acid bacteria (LAB) and applying them in a food product is a challenge due to the large sizes of bacterial cells that lead to the production of large beads [Mahmoud et al., 2020].
Table 2
Average particle diameter (d3,2, μm) of capsules of Lactiplantibacillus plantarum and beetroot extract with alginate obtained by different encapsulation techniques.
Needle caliber | Extrusion | Emulsion |
---|---|---|
G31×6mm | 978±42e | 600±150f |
G27×13mm | 1,250±37c | 1,050±120d |
G22×32mm | 2,260±120b | 2,850±260a |
Figure 1
Morphology of the alginate beads loaded with Lactiplantibacillus plantarum co-encapsulated with beetroot extract obtained by extrusion (A-C) and emulsion (D) techniques using different needle calibers: G22×32 mm (A), G27×13 mm (B), G31×6 mm (C), G31×6 mm (D). Magnification was 10×. The measurements of the capsules were obtained with the Image-Pro Plus 2D analysis software with the caliper tool.
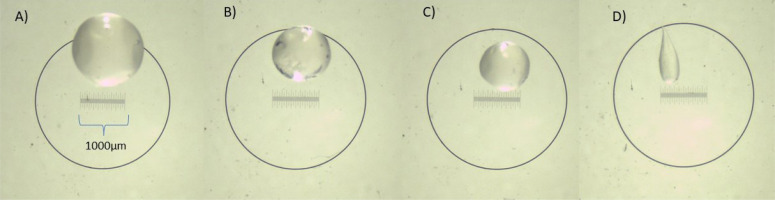
The variability in the beads’ morphology could affect the encapsulation efficiency and the results could hardly be replicated. Due to the variability in size and morphology of the beads obtained by the emulsification, we selected the extrusion technique for further analyses. The smallest bead sizes produced by this last method were for the needle with the caliber of G31×6mm, thus it was chosen for bead production.
Encapsulation efficiency and viability of L. plantarum after 24 h
The counts of encapsulated L. plantarum (natural beads, NB) and L. plantarum co-encapsulated with beetroot extract (beetroot beads, BB), the count of bacteria in both types of beads after 24 h, as well as encapsulation efficiency (%EE) and percentage of bacteria viability after 24 h (%V24h) are presented in Table 3. The counts of encapsulated cells were 7.59 and 7.82 log cfu/g for NB and BB, respectively, with an encapsulation efficiency of 86.4–88.0%. No significant differences (p≥0.05) in %EE were evidenced between the different formulations when cells were incorporated. These results are in agreement with the study by Pupa et al. [2021], who reported an efficacy of alginate – L. plantarum encapsulation in the range from 73.64 to 94.10%. They found a higher efficiency in the extrusion method compared to the spray drying method. Mahmoud et al. [2020] reported an efficiency of up to 94% with no significant differences between different encapsulating agents (skim milk, dextrin and chitosan) in combination with the alginate loaded with L. plantarum. In addition, our results showing that the content of beetroot extract did not affect the %EE are in line with the literature data; according to Krasaekoopt & Watcharapoka [2014], the effectiveness of encapsulation is independent of the concentration of prebiotics incorporated. Otherwise, the encapsulated bacteria viability after 24 h (%V24h) varied among formulations with better survival noted in beetroot beads (Table 3). A significantly (p<0.05) lower bacterial count by 1.52 log cfu/g was evidenced after 24 h in NB. The much smaller loss of 0.54 log cfu/g was noted for BB. This last assay is important to determine any negative effect of the beetroot extract on L. plantarum cells. A significant decrease within 24 h in the number of bacteria co-encapsulated with beetroot extract could indicate the occurrence of antimicrobial activity; if that is the case, co-encapsulation would not be feasible. On the contrary, if the population is maintained or increased, we can infer that the presence of the beetroot extract does not exert a negative effect or that it may actually be beneficial for the bacteria. Our results indicate that the incorporation of the beetroot extract in the alginate beads does not exert negative effects on L. plantarum. Since L. plantarum showed higher viability after 24 h in BB than in NB (Table 3), the effect of beetroot extract incorporation was found positive. According to Shafizadeh et al. [2020], some compounds co-encapsulated with bacteria could confer certain protective effect. Other investigations reported that the viability of encapsulated probiotics was improved when adding prebiotics, i.e., raftilose [Capela et al., 2006], inulin [Akhiar, 2010], galacto-oligosaccharides and fructo-oligosaccharides [Amine et al., 2014], as well as dextrin and whey [Mahmoud et al., 2020] among others. These previous reports are consistent with our results, in which the V24h was higher in BB than NB. Based on this literature data and our results, it can be concluded that the beetroot extract acted as a prebiotic.
Table 3
Encapsulation efficiency (%EE) of Lactiplantibacillus plantarum with alginate (natural beads, NB) and L. plantarum and beetroot extract with alginate (beetroot beads, BB), percentage of bacteria viability after 24 h (%V24h) and percentage of bacteria survival after incubation under simulated gastrointestinal conditions.
The results presented in Table 3, show that the encapsulation efficiency was less than 95% for the beads produced. Even though other investigations have reported %EE above 95%, this could be improved by increasing the number of initial cell counts [Pupa et al., 2021].
Viability of L. plantarum under simulated gastrointestinal conditions
The survival percentages of L. plantarum encapsulated in NB and BB after the incubation under simulated gastrointestinal conditions are shown in Table 3. For this assay, the free L. plantarum was used as a respective control. Encapsulated L. plantarum had better viability compared with the free cells, which were not detectable at the end of the process. These results are in agreement with those reported by Khosravi et al. [2014], who found no survival of free Bifidobacterium bifidum after exposure to simulated gastrointestinal conditions. Several other studies have reported better survival of bacteria in the capsules than in the non-encapsulated form, during exposure to unfavorable conditions [Chávarri et al., 2010; Khosravi et al., 2014; Krasaekoopt & Watcharapoka, 2014; Mahmoud et al., 2020; Pupa et al., 2021]. Besides this, significant difference (p<0.05) in the survival percentage was evidenced between the NB and BB, proving that BB ensured a better cell survival rate (73.3%) when compared with NB (57.4%) (Table 3). The losses of bacteria were 3.1 and 2.0 log cfu/g for NB and BB, respectively. This could suggest that beetroot had a protective effect on L. plantarum, which allows major survival of the bacteria in the gastric environment, compared with the bead that does not contain beetroot. Other studies reported a protective effect generated by the incorporation of skim milk [Mahmoud et al., 2020] or amylomaize [Khosravi et al., 2014], with losses ranging from 2.5 to 4.6 log cfu/g after exposure to gastrointestinal conditions, depending on the bacteria and the co-encapsulated material. It has been reported that beetroot represents a rich source of bioactive compounds and contains certain amounts of nutrients (i.e., carbohydrates and proteins) that could protect the bacterial cells and thus maintain their viability [Barbu et al., 2020]. Although it has been reported that buffering capacity of some ingredients can protect probiotic bacteria in the product or during intestinal transit [Shori, 2017; Tripathy & Giri, 2014], with the data presented here we cannot ensure which compound of beetroot was specifically responsible for this protection. According to Mahmoud et al. [2020], this protection may be influenced by the strong structure of the bead, or the nutritional factor conferred by the incorporation of beetroot extract.
Effect of the addition of capsules on the rheological properties of model food
To obtain functional food with capsules, different formulations of dressings based on O/W emulsion were prepared (Table 1). For monitoring the stability of the products, flow properties, the droplet size and coalescence rate were monitored during storage. The dressing without the addition of beads (DWB) was used as the control.
Flow properties of dressings
The flow behavior of O/W emulsions is important because the behavior of different formulations can be compared and appropriate process conditions, as well as optimal quality control strategies may be chosen accordingly [Mun et al., 2009]. In our study, to evaluate changes in viscosity of dressings over time, emulsion flow curves (the changes in apparent viscosity as a function of shear rate) were obtained at the initial time of storage (zero time) and at the end of storage (28 days). Flow curves are presented in Figure 2. The behavior of the different dressings was consistent with the power law. Overall, dressings with NB and BB added exhibited non-Newtonian stress thinning behavior and a creep point related to the systems’ initial resistance to flow. The control sample and formulated dressings showed a similar trend. To identify the flow characteristics of the dressings, the values of consistency coefficient (k) and flow behavior index (n) were obtained as a function of the shear rate. The k is often used as an indicator of fluid viscosity, which is a measure of the fluid’s ability to resist deformation; high values indicate high viscosity and a stronger and more stable network structure [Castillo et al., 2021]. When evaluating the flow behavior index, a value less than 1 represents pseudoplasticity; this characteristic is desirable for obtaining a dressing with a good mouth feel [Ma & Boye, 2013; Primacella et al., 2019]. On the other hand, a high k value is desirable for the stability of the system. The k and n values for the formulated dressings (DWB, DNB, and DBB) at zero time and after 28 days of storage are shown in Table 4. Additionally, a commercial dressing (COD) was analyzed as a point of comparison. The initial k values varied from 12.52 to 14.52 Pa×sn, with no significant differences (p≥0.05) among the formulations prepared, including the COD, indicating a similar viscosity of all the formulations tested. When the initial flow behavior index was considered, low values (<1) were evidenced for all the formulations. The initial n values varied from 0.239 to 0.299. Significant difference (p<0.05) was noted only between the DWB and COD formulations. Anyway, there was n<1 for all the formulations, so we could expect a good texture of the product. After 28 days of storage, the k values ranged from 13.93 to 17.23 Pa×sn. On the other hand, the n values ranged from 0.249 to 0.300. The results showed no significant changes (p≥0.05) in k and n values over the storage period (after 28 days) regardless of the formulation, which indicates that the addition of the capsules did not affect the rheological properties of the system.
Table 4
Consistency coefficient (k) and flow behavior index (n) of dressings at the initial (day 0) and final time (28 days) of storage, and coalescence rate (Kc) of model food.
[i] Results are expressed as mean of three repetitions ± standard deviation. Values with different minor letters (a–c) in the same column are significantly different (p<0.05). Values with different capital letters (A, B) in the same row for each parameter separately (k or n) are significantly different (p<0.05). COD, commercial dressing; DWB, dressings without beads; DNB, dressing with encapsulated Lactiplantibacillus plantarum with alginate (natural beads); DBB, dressing with co-encapsulated L. plantarum and beetroot extract with alginate (beetroot beads).
Figure 2
Apparent viscosity as a function of shear rate of the dressings at the initial (A) and final time of storage (28 days) (B). DWB, dressing without beads; DNB, dressing with encapsulated Lactiplantibacillus plantarum with alginate (natural beads); DBB, dressing with co-encapsulated L. plantarum and beetroot extract with alginate (beetroot beads); COD, commercial dressing.
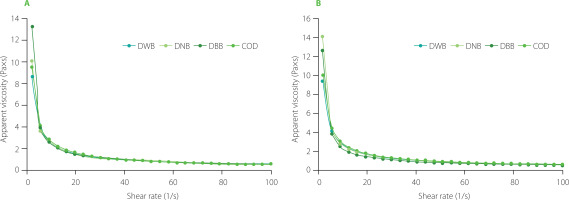
Droplet size and coalescence rate of dressings
An incorporation of a new ingredient can destabilize the system and may affect shelf life. Stability of the system can be monitored through changes in the average particle diameter over time and also implies prevention of coalescence [Castillo et al., 2021]. Averages of droplet size (d3,2) of the formulations were measured at zero time and after 14 and 28 days of storage. Changes in droplet size varied along time in the three formulations (Table 5). A significant difference (p<0.05) was found on day fourteen of storage only for DNB (d3,2=4.380) compared with DWB (d3,2=3.942) and DBB (d3,2=3.836). On the other hand, on day 28 of storage, significant differences (p<0.05) were found for DNB (d3,2=4.326) and DBB (d3,2=3.925) compared with DWB (d3,2=3.488). Despite the differences in those points, no significant differences (p≥0.05) were found in the global behavior throughout the 28 days.
Table 5
Average particle diameter (d3,2, μm) of the dressings at 0, 14 and 28 days of storage.
Dressing | 0 day | 14 days | 28 days | Mean |
---|---|---|---|---|
DWB | 3.514±0.011a | 3.942±0.115b | 3.488±0.015b | 3.624±0.215a |
DNB | 3.518±0.013a | 4.380±0.027a | 4.326±0.053a | 4.070±0.408a |
DBB | 3.512±0.007a | 3.836±0.025b | 3.925±0.041a | 3.786±0.217a |
[i] Results are expressed as mean of three repetitions in triplicate ± standard deviation. Different letters (a,b) in the same column represent significant differences (p<0.05). DWB, dressings without beads; DNB, dressing with encapsulated Lactiplantibacillus plantarum with alginate (natural beads); DBB, dressing with co-encapsulated L. plantarum and beetroot extract with alginate (beetroot beads).
Another parameter that provides important information about stability of the emulsion is the coalescence rate (Kc), which can be measured through changes in the average diameter of the drops over time. This parameter allows the collection of quantitative data on system stability. The coalescence rate of the different formulations is reported in Table 4. Despite a significant difference (p<0.05) was noted for DWB (−4×10−8) compared with the other formulations, the coalescence rate for all the dressings tested had very low values with exponents −7 and −8. The formulations with encapsulated probiotic showed similar behavior compared with the COD with values ranging from −1×10−7 to −3×10−7. According to Bautista et al. [2018], values at this level indicate a stable system. When the droplet coalescence rate is high, a decrease in the emulsion’s viscosity may occur because the coalescence of emulsions is inversely related to the viscosity of the aqueous phase [Krstonošić et al., 2009]. We can observe in our results that k of the formulations did not decrease during storage (Table 4).
The coalescence of emulsions in storage conditions are influenced by different factors, as collision frequency and drainage time [Ye et al., 2004]. This phenomenon could take place in different stages of emulsion formulation, i.e., whipping process or storage [Petrut et al., 2016]. The addition of certain ingredients including those with different functional characteristics could cause changes in stability. Preserving the original viscoelastic properties is very important to the quality of the product that is strongly influenced by its rheological properties and appearance attributes [Ma & Boye, 2013]. Losses in stability provoked by the increase in droplet mean diameter of the emulsion shorten the shelf life. Therefore, obtaining a product with an extended shelf life is a challenge during food product formulation [Traynor et al., 2013].
Viability of encapsulated L. plantarum in a model food in storage conditions
The NB and BB loaded with L. plantarum were added to a dressing (DNB and DBB, respectively) and the viability of the probiotic was determined over time. Viable counts of L. plantarum during 28 days of storage at 4°C are presented in Figure 3A. Also, the percentage of viability was calculated and results are shown in Figure 3B. Even though the number of viable cells of L. plantarum decreased as time progresses in both models, the DBB maintained a greater viability of the probiotic over time, ranging from 7.09 to 4.3log cfu/g, and a percentage of viability ranging from 94.4% to 60.6% along the storage time, respectively. On the other hand, the levels of cells recovered for the DNB were significantly lower (p< 0.05) from the 8th day on. The bacterial population decreased from 6.90 to 2.1 log cfu/g at the end of storage for this last model, while the percentage of viability ranged from 79.2% to 30.6%, respectively. At the end of storage, a total diminution of 2.8 log cfu/g was detected for DBB and 4.8 log cfu/g for DNB, suggesting that encapsulated beetroot could act as a prebiotic ingredient. Our results are in agreement with Chavarri et al. [2010], who reported losses of encapsulated probiotic bacteria ranging from 2.1 to 3.4 log cfu/g along the time of storage (28 days), depending on the bead matrix. Additionally, Rodríguez Huezo et al. [2011] reported losses of 4.0 log cfu/g for encapsulated probiotics without prebiotic ingredient in a period of 25 days.
Figure 3
Viable counts of encapsulated Lactiplantibacillus plantarum along time of storage at 4°C in the model food (A). Percentage of viability of L. plantarum along storage time in the model food (B). Error bars represent standard deviation. Different letters in the top of the bars (a–d) represent significant differences (p<0.05). DNB, dressing with encapsulated L. plantarum with alginate (natural beads); DBB, dressing with co-encapsulated L. plantarum and beetroot extract with alginate (beetroot beads).
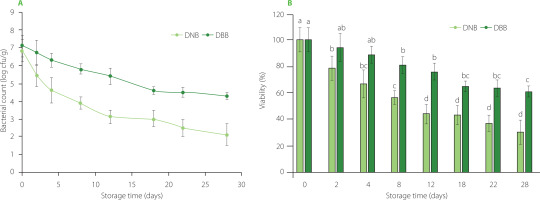
Although the population of L. plantarum continued to decline by the 28th day, the population remained over 60% in DBB, while the DNB model had less than 35% of viability at the end of storage. These results are consistent with other reports, in which greater viability of probiotics was observed when encapsulated with prebiotics rather than with natural beads [Capela et al., 2006; Krasaekoopt & Watcharapoka, 2014; Mahomud et al., 2020]. Krasaekoopt & Watcharapoka, [2014] reported greater viability of L. casei and L. acidophilus encapsulated with galacto-oligosaccharides compared with those without this prebiotic. The authors also reported minimal losses of population (0.5 to 2.5 log cfu/g) along four weeks of storage. Additionally, Mahomud et al. [2020] reported better survival in storage conditions for L. plantarum when co-encapsulated with skim milk, and in some cases, an increase in population was evidenced. According to Barbu et al. [2020], diverse factors may influence the growth of the probiotics; in these cases, it could be attributed to biocomposites and nutrients from the bead matrix that somehow protect the bacterial cells, and thus maintain their viability. In a dressing, a probiotic bacterium must be kept immobilized to prevent proliferation, and in this way prevent food decomposition; when encapsulating the bacteria, it remains immobilized, protecting the integrity of the food while maintaining its attributes, such as pH and texture. Likewise, it has been demonstrated that beetroot consumption has a beneficial effect on the gut microbiota in humans, induced by a prebiotic effect [Alexandrino de Oliveira et al., 2021]. Our results have shown a higher microbial recovery during storage time in the DBB model, so this model could have been influenced by the presence of the beetroot extracts inside the capsules.
Industrially, encapsulated probiotic bacteria can be used in many products (i.e., yogurt, cheese or frozen dairy desserts). This strategy provides higher stability of bacteria also protecting the components incorporated against nutritional loss [Anal & Singh, 2007; Khosravi et al., 2014].
CONCLUSIONS
The extrusion technique demonstrated greater feasibility compared to emulsification for obtaining capsules of L. plantarum with alginate and L. plantarum and beetroot extract with alginate with homogeneous morphology and size. The co-encapsulation of L. plantarum and beetroot extract with alginate conferred better protection for bacteria under gastrointestinal conditions than the encapsulation of L. plantarum along with alginate Although the protection exerted by beetroot on the bacteria was evidenced, due to the number of components that beetroot contains, it is difficult to determine the driving mechanisms of this protection. Further studies are ongoing on this aspect. When beads were incorporated to the O/W emulsion model food, they did not cause changes in the flow properties or droplet coalescence, indicating that the addition of the capsules does not cause system destabilization. Additionally, beetroot beads preserved the viability of L. plantarum during 28 days of storage in the product, indicating that the incorporation of beetroot in the capsule could have a prebiotic effect on L. plantarum. Future studies should be carried out to evaluate the acceptability of the developed product.