INTRODUCTION
The lipid content and composition of marine organisms, including fish, mollusks, and various other marine species, exhibit significant variability influenced by factors such as species, season, gender, and geographical location [Kandemir & Polat, 2007; Nguyen et al., 2024]. The lipid content is also influenced by the dietary nutritional profile [Anjos et al., 2017]. In our previous study, we demonstrated that Pacific oysters (Crassostrea gigas) cultured in Khanh Hoa Coast, Vietnam, were rich in exploitable lipids, particularly polyunsaturated fatty acids (PUFAs), and their composition varied with season and location [Nguyen et al., 2024]. Oyster lipids, comprising a mixture of neutral and polar lipids, play essential roles in biological functions and exhibit bioactivities with potential health benefits [Tan et al., 2022]. Neutral lipids primarily consist of triacylglycerols and wax esters, while polar lipids are composed of phospholipids and glycolipids. The structure and function of polar and neutral lipids vary among different species [Şen Özdemir et al., 2019]. Understanding the specific fatty acid composition and potential health implications of these lipids is crucial for exploring their nutritional and pharmaceutical potential.
Studies have shown that extracts from a range of marine organisms, including mollusks, possess bioactivities with potential health benefits, including antiviral activity, antioxidant, antiinflammatory, immunomodulatory, and anticancer properties [Hamed et al., 2015; Khan & Liu, 2019]. The active compounds in these extracts include proteins and glycoproteins (active against viruses) [Dang et al., 2015], and PUFAs with reported anti-inflammatory properties among others. Marine-derived lipids have been demonstrated to exhibit anticancer activity in various studies [Lauritano et al., 2020; Li et al., 2020; Martínez Andrade et al., 2018]. Similarly, extracts from marine microorganisms and algae have shown potential as novel anticancer agents, highlighting the abundance of bioactive compounds present in marine ecosystems [Samarakoon et al., 2014; Tommonaro et al., 2020]. Kim et al. [2010] demonstrated that the hexane lipid extract from C. gigas exhibited growth-inhibitory activity against the prostate cancer cell line. This lipid fraction was comprised of palmitic, margaric, and stearic acids. In a study by Nappo et al. [2012] conducted with marine diatoms Cocconeis scutellum Ehrenberg (Bacillariophyceae), the diethyl ether extracts rich in EPA induced apoptosis and decreased viability in BT20 cells. Oyster lipids contain several glycerophospholipids with triacylglycerols containing n3 long chain-PUFAs that are presumed to have better tissue delivery capacity and bioavailability [Liu et al., 2020].
It is important to find new sources of bioactive compounds which are useful in the development of therapeutic agents for cancer treatment. Challenges can be encountered in harvesting potential organisms and isolating and purifying the bioactive compounds, which call for more organisms to be explored. Investigation into the fatty acid composition and anticancer properties of oyster lipids, particularly those cultured in diverse geographical locations such as the Nha Phu Lagoon, Khanh Hoa Coast, is useful in this scenario.
In this study, we investigated the fatty acid composition and potential anticancer activity of neutral and polar lipids extracted from Pacific oysters cultured in the Nha Phu Lagoon, Khanh Hoa Coast, Vietnam harvested during January, April, May, September, and November. Specifically, our study aimed to assess the effects of oyster lipids on three human cancer cell lines: MDA-MB-231 (human breast cancer), HepG2 (liver cancer), and RD (muscle rhabdomyosarcoma-A) cell lines.
MATERIALS AND METHODS
Materials and reagents
Sample collection and preparation
Commercial-quality Pacific oysters (Crassostrea gigas) were obtained from Nha Phu Lagoon, located in Ninh Hoa District, Khanh Hoa Province, Vietnam, during multiple harvests conducted in January, April, May, September, and November 2021. The sample collection and preparation were carried out following the procedure described by Nguyen et al. [2024]. Briefly, the oyster muscle was separated from the shells, vacuum-sealed in polyamide bags, and promptly frozen at −35±2°C using an air-blast freezer (Seatecco Corporation, Da Nang, Vietnam). Frozen samples were stored at −80°C and thawed completely at 2°C before analysis.
Analytical materials and reagents
Analytical-grade materials and reagents including n-hexane, chloroform, methanol, l-ascorbic acid, thin-layer chromatography (TLC) plates (TLC silica gel 60 F254), silica gel 60 (0.040–0.063 mm), diethyl ether, potassium chloride, sodium sulfate, trypan blue, and ninhydrin were procured from Sigma-Aldrich (Burlington, MA, USA). Additionally, l-glutamine, penicillin-streptomycin, trypsin-EDTA, Dulbecco’s modified Eagle medium (DMEM), minimum essential medium (MEM), and fetal bovine serum (FBS) were obtained from Gibco (Billings, MT, USA). Doxorubicin was sourced from EBEWE Pharma (Unterach am Attersee, Austria), while all other reagents were of analytical grade and were purchased from Merck (Darmstadt, Germany). Human cell lines, including MDA-MB-231 (human breast cancer), HepG2 (liver cancer), and RD (muscle rhabdomyosarcoma-A), were procured from ATCC (Manassas, VA, USA).
Lipid extraction and fractionation
Total lipids were extracted from oyster muscle following the method of Bligh & Dyer [1959]. Briefly, oyster muscle was homogenized using an Ultra-Turrax homogenizer (T25 basic, Ika Labortechnik, Staufen, Germany) in a mixture of chloroform, methanol, and 0.88% KCl (1/1/0.5, v/v/v). The homogenate was then subjected to centrifugation at 1,942×g (Hermle Z326K universal refrigerated centrifuge, Wehingen, Germany) for 20 min at 4°C. The lipid layer was collected, and the chloroform was completely evaporated at 40°C in a water bath using a liquid nitrogen stream. Subsequently, total lipids were separated into neutral and polar lipid fractions via column chromatography on silica gel, following the procedure described in our earlier work [Nguyen et al., 2024]. A solution containing 0.8 g of the lipid sample in 1 mL of chloroform was loaded onto a column (180 mm in diameter and 330 mm in length) filled with silica gel 60 (0.040–0.060 mm). The neutral lipid fraction was obtained by eluting with a chloroform-methanol solvent. The polar lipid fraction was recovered by elution with methanol. The complete recovery of the neutral and polar lipid fractions was confirmed using thin-layer chromatography (TLC) [Deranieh et al., 2013]. The larger volumes of the solvents of each fraction were removed under vacuum at 40°C (Yamato RE-801-AW2 rotary evaporator, Yamato, Japan). The residual solvent was evaporated under a stream of nitrogen at 35°C. The obtained lipid fractions were used for the analysis of fatty acid composition and anticancer activity.
Fatty acid composition analysis
Fatty acid methyl esters (FAMEs) of neutral and polar lipid fractions were prepared via base-catalyzed esterification according to the American Oil Chemists’ Society (AOCS) official method (Ce 1b-89, 2017) [AOCS, 2017]. Subsequently, the FAMEs were subjected to gas chromatography (GC) analysis using a Shimadzu GC 17A chromatograph (Shimadzu Corp., Kyoto, Japan) with flame-ionization detector. Separation was carried out on a Zebron ZB-wax column (0.25 mm × 30 m, 0.25 μm; Phenomenex, Torrance, CA, USA). The initial temperature of column oven and injection port was 170°C. This temperature was maintained for 2 min, then increased to 240°C at a rate of 5°C /min, from 240°C to 250°C at a rate of 1.6°C /min, and finally held at 250°C for 10 min. The inlet pressure of nitrogen, which was used as a carrier gas, was 2.0 kg/cm2. Results were expressed as g per 100 g of the lipid fraction (g/100 g LF), and tricosanoic acid (C23:0) was used as an internal standard.
Thrombogenicity index calculation
The thrombogenicity index (TI) was computed according to the equation proposed by Ulbricht & Southgate [1991], which evaluates the relationship between pro-thrombogenic saturated fatty acids and anti-thrombogenic monounsaturated fatty acids (MUFAs) and PUFAs.
Determination of anticancer activity
The assessment of the anticancer properties of polar and neutral lipid fractions of C. gigas oysters harvested in January followed the protocol outlined by Tran et al. [2020], with modifications. MDA-MB-231, HepG2, and RD cell lines were cultured in EMEM and DMEM media. The lipid fractions were initially dissolved in dimethyl sulfoxide, DMSO (10 mM) and subsequently diluted in the culture medium to obtain various concentrations of 6.25, 12.50, 25.00, 50.00, and 100.00 μg/mL for the cell proliferation assays. Following treatment with lipid fractions at varying concentrations, cells were incubated for 72 h at 37°C and 5% CO2. Cell viability was determined using 3-(4,5-dimethylthiazol-2-yl)-2,5-diphenyltrazolium bromide (MTT) assay as outlined by Denizot & Lang [1986]. Doxorubicin was employed as the reference compound, while DMSO served as the blank control. IC50 values, representing the concentrations of lipid fractions that inhibit 50% of cell viability, were determined based on the dose-response inhibition curves. The inhibition rates of the neutral and polar lipid fractions against the three tested cancer cell lines were calculated using the following 2:
where: ODSample is optical density of the final assay mixture with lipid fraction, ODDMSO is optical density of the final assay mixture with DMSO.Statistical analysis
The experiments were performed in triplicate, and the data were expressed as mean values and standard deviations (SD). Statistical analyses were conducted using the SPSS software (version 26, SPSS Inc., Chicago, Il, USA). One-way analysis of variance (ANOVA) was employed to analyze the results. Significance of differences between the samples was determined using the Student-Newman-Keuls post-hoc test at a significance level of 5%.
RESULTS AND DISCUSSION
Fatty acid composition in the neutral lipid and polar lipid fractions
The changes in fatty acid composition of the neutral and polar lipid fractions in C. gigas oysters cultured in Nha Phu Lagoon, Khanh Hoa Coast, Vietnam, for the months of January, April, May, September, and November are presented in Table 1. Neutral lipids extracted from C. gigas were abundant in SFAs, followed by PUFAs, and MUFAs. The SFAs content of the neutral lipid portion increased from January (46.94 g/100 g LF) to a maximum in September (53.35 g/100 g LF) and then decreased in November (47.17 g/100 g LF). The SFAs content was significantly different (p<0.05) for the five months. The MUFAs content of the neutral lipid fraction showed slight variation, with the highest content recorded in the month of May (22.91 g/100 g LF), while the lowest was in the month of September (19.66 g/100 g LF). The MUFA content of the neutral lipid fraction for the months of January, April, and May was not significantly (p<0.05) different from each other, but significantly different (p<0.05) from that obtained in September. The major MUFAs in neutral oyster lipids was myristoleic acid (C14:1n9), while palmitic acid (C16:0) was identified as the predominant saturated fatty acid (SFA). The PUFAs content was relatively stable in January, April, and November and only decreased (p<0.05) during May and September. The main PUFAs in the neutral lipids were the n3 PUFAs with eicosapentaenoic acid (EPA, C20:5n3, 5.12–7.94 g/100 g LF) and docosahexaenoic acid (DHA, C22:6n3, 5.52–9.61 g/100 g LF). The EPA and DHA contents were significantly (p<0.05) lower in the months of May and September. In contrast to neutral lipids, polar lipids exhibited a higher abundance of PUFAs (36.12–47.22 g/100 g LF), followed by MUFAs (27.68–33.64 g/100 g LF), and then SFAs (20.74–30.52 g/100 g LF). The oysters harvested in May and September had lower (p<0.05) PUFA contents compared to those harvested in January, April, and November. These findings were consistent with the higher SFA contents determined in the oysters harvested in May and September. The PUFAs content of the polar lipids was over 40 g/100 g LF except in the months of May and September, where it was approximately 36 g/100 g LF. Similar to the neutral lipid fraction, EPA and DHA were the dominant PUFAs; however, their levels in the polar lipid fraction were significantly higher (p<0.05) compared to those found in the neutral lipids. In the polar lipid fraction, oleic acid (C18:1n9) was the predominant MUFA, whereas palmitic acid (C16:0) remained the most abundant SFA. The total DHA and EPA content was over 30 g/100 g LF for all the months. The PUFA content in the polar lipid fraction was significantly (p<0.05) higher than that of the neutral lipid fraction. Glycolipids and phospholipids are typically esterified with EPA [Da Costa et al., 2021], and marine polar lipids play a crucial role as carriers of n3 fatty acids, containing higher levels of n3 PUFAs compared to triglycerides [Lordan et al., 2011; 2017]. The observed increase in the SFAs content of the neutral lipid fraction during the months of May and September (spawning season) may be attributed to the elevated energy demands necessary during this period. The increased levels of PUFAs in January and November align with phytoplankton blooms during the rainy seasons. Since PUFAs have a high tendency to be esterified to phospholipids rather than triacyclglycerols, their content increases in phospholipids during gonadal maturation. PUFAs serve a significant role in the composition of structural membrane lipids and are stored as lipovitellins in oocytes. These lipovitellins act as reserves for the cellular division process that occurs after fertilization [De La Parra et al., 2005]. Palmitic acid, EPA, and DHA were identified as principal constituents of phospholipids, which are characteristic of marine animals [Pogoda et al., 2013]. Previous studies have consistently demonstrated that oysters are rich sources of EPA and DHA [Liu et al., 2020; Martino & Cruz, 2004]. High levels of EPA are indicative of the presence of EPA-rich phytoplankton. Previous studies have also revealed the energetic role of EPA [Qin et al., 2021]. In mollusks, the fatty acid C20:4n6 acts as a precursor for prostaglandins, which play a crucial role in the regulation of reproductive processes [Soudant et al., 1999]. It has been suggested that marine bivalves exhibit a greater need for accumulating n3 PUFAs compared to n6 PUFAs [Abad et al., 1995]. This observation elucidates the higher levels of n3 PUFAs compared to n6 PUFAs discovered in our study. Pogoda et al. [2013] similarly reported that the fatty acid composition of Ostrea edulis and C. gigas was predominantly composed of C16:0, EPA, and DHA, consistent with the findings of our study. The fatty acid composition of oysters is influenced by both intrinsic factors such as sex, age, and size as well as extrinsic factors including temperature, salinity, and diet [Martino & Cruz, 2004].
Table 1
Fatty acid composition (g/100 g lipid fraction) of neutral and polar lipid fractions of Crassostrea gigas oysters cultured in Nha Phu Lagoon, Khanh Hoa Coast harvested during different months
Nutritional quality of neutral and polar lipid fractions
The changes in thrombogenicity index (TI) and an n3/n6 ratio of neutral and polar lipids of oyster harvested in different months are shown in Figure 1. A high ratio of n3/n6 is necessary and important in marine organisms for growth and survival [Soudant et al., 1999]. Significantly higher n3/n6 ratios were observed in the polar lipid fraction compared to the neutral lipid fraction (Figure 1A). In the polar lipid fraction, the n3/n6 ratio exhibited a significant decline from January to September, followed by a significant increase in November. Notably, the n3/n6 ratios of polar lipids extracted from oysters harvested in May and September were significantly lower (p<0.05) compared to the ratios observed in other months. The observed reduction in n3/n6 ratios during May and September could be attributed to lipid accumulation and the conversion of glycogen into neutral lipids that occur during the spawning season. The pattern of changes in the n3/n6 ratio of the neutral lipid fraction was similar, but no significant (p≥0.05) differences were found in the n3/n6 ratio among oysters harvested in different months. Diets with low n3/n6 ratios are associated with an increase in inflammatory diseases [Qin et al., 2021]. Therefore, the consumption of polar lipids from oysters is crucial for maintaining good health, as they contain elevated levels of omega-3 fatty acids in comparison to neutral lipids.
Figure 1
Variations in n3/n6 ratio (A) and thrombogenicity index, TI (B) of neutral and polar lipids of Crassostrea gigas oysters cultured in Nha Phu Lagoon, Khanh Hoa Coast harvested during different months. Different letters above bars separately for each lipid fraction indicate significant differences (p<0.05).
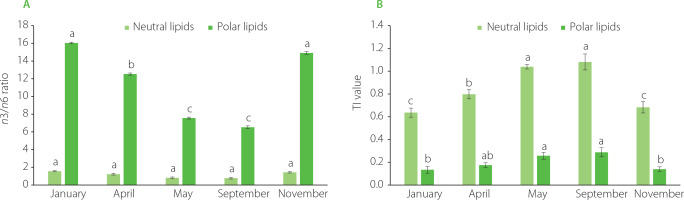
The TI for both the neutral and polar lipid fractions gradually increased from January to September and then decreased in November (Figure 1B). The TI values for the neutral lipid fraction were significantly higher (p<0.05) than those of the polar lipid fraction. TI values for the polar lipid fraction ranged between 0.13 and 0.29, while those for the neutral fraction ranged between 0.6 and 1.1. The TI is associated with the risk of thrombosis, and its values exceeding 1.0 are considered hazardous to human health [Chakraborty et al., 2016]. High TI values were observed for the neutral lipid fraction in May and September, reaching 1.0 and 1.1, respectively. These elevated TI values are attributed to the increased content of saturated fatty acids during the spawning season. As spawning requires significant energy, oysters utilize their lipid reserves to meet these demands. Since polar lipids contain a higher proportion of PUFAs, the TI values for the polar lipid fraction are significantly lower than those of the neutral lipid fraction.
Anticancer activity of neutral and polar lipid fractions
The anticancer activity of the polar and neutral lipid fractions extracted from C. gigas was evaluated against human breast cancer (MDA-MB-231), liver cancer (HepG2), and muscle rhabdomyosarcoma-A (RD) cell lines using the MTT assay. Both the polar and neutral lipid fractions of C. gigas exhibited weak inhibitory effects on the cell growth of the three tested cell lines. The extent of this effect was dependent on the concentration of the lipid fractions. After 72 h of treatment with the neutral and polar lipid fractions at a concentration of 100 µg/mL, the inhibition rates of the polar lipid fraction on the proliferation of HepG2 and RD cells were significantly (p<0.05) higher than those of the neutral lipid fraction. Inversely, the inhibition rate of the neutral lipid fraction on the proliferation of MDA-MB-231 cells was significantly (p<0.05) higher compared to that of the polar lipid fraction (Figure 2). For the polar lipid fraction, the inhibitory effect on cell proliferation decreased gradually in the following order: HepG2 cells>MDA-MB-231 cells>RD cells. In terms of the neutral lipid fraction, the inhibitory effect on the proliferation of the three tested cell lines decreased gradually in the following order: MDA-MB-231 cells>HepG2 cells>RD cells. After a 72-h treatment, the IC50 values of the polar and neutral lipid fractions against the three tested cell lines were both found to be above 100 µg/mL (Table 2). In comparison, the IC50 values of the doxorubicin positive control were 0.60 µg/mL, 1.35 µg/mL, and 1.40 µg/mL for MDA-MB-231, HepG2, and RD cells, respectively. The results obtained regarding the cell growth inhibitory effects of the polar and neutral lipid fractions against the three tested cell lines corresponded to the morphological changes observed in cancer cells treated with the studied samples, as examined through phase contrast microscopy (Figure 3). It is worth noting that a previous study conducted by Wang et al. [2017] reported similar findings. In their study, the IC50 value of the polar lipid fraction extracted from the brain of silver carp against MCF-7 cells was significantly lower compared to that of the total lipid and neutral lipid fractions. Additionally, consistent with our study, the IC50 values of all lipid fractions in their research exceeded 100 µg/mL. The IC50 values of the neutral lipid extracted from Scylla paramamosain against five cancer cell lines, including SK-LU-1 cells, HL-60 cells, HT-29 cells, HepG2 cells, and MCF7 cells, were found to be above 100 µg/mL, whereas the IC50 values of the polar lipid fraction ranged from 85.4 to 95.8 μg/mL [Nguyen et al., 2020]. The difference in inhibitory activity of lipid fractions against various cell lines can be attributed to variations in the fatty acid composition of the lipid extract [Nappo et al., 2012; Wang et al., 2014].
Table 2
Anticancer activity of neutral and polar lipid fractions of Crassostrea gigas oysters against cancer cell lines.
Lipid fraction | IC50 (µg/mL) | ||
---|---|---|---|
MDA-MB-231 | RD | HepG2 | |
Neutral lipids | >100 | >100 | >100 |
Polar lipids | >100 | >100 | >100 |
Doxorubicin* | 0.60±0.04 | 1.40±0.10 | 1.35±0.04 |
Figure 2
Inhibition rates against MDA-MB 231, RD and HepG2 cell lines treated at a concentration of 100 µg/mL for 72 h with neutral and polar lipids of Crassostrea gigas oysters cultured in Nha Phu Lagoon, Khanh Hoa Coast. Different letters above bars separately for each cancer cell line indicate significant differences (p<0.05).
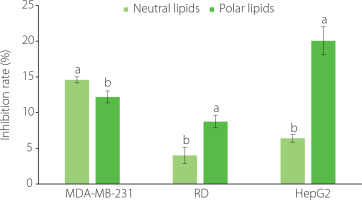
Figure 3
Morphological changes in MDA-MB 231, RD and HepG2 cell lines treated with neutral lipid and polar lipid fractions of Crassostrea gigas oysters cultured in Nha Phu Lagoon, Khanh Hoa Coast.
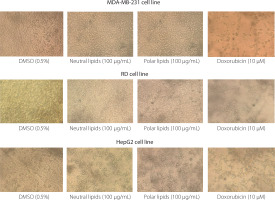
Previous studies have demonstrated that PUFAs show cytotoxicity in cancer cells [De Gaudry et al., 2014; Dekoj et al., 2007; Kang et al., 2010], and supplementation with DHA has been shown to significantly enhance apoptosis [Das & Das, 2016]. In this study, we observed that the cytotoxicity of the lipid fractions in the three cancer cell lines was relatively low. Nonetheless, the polar lipid fraction exhibited promising cytotoxic activity specifically against HepG2 cells. Similar findings have been reported by Nguyen et al. [2020], where polar lipids derived from crabs exhibited cytotoxic activity against five different cancer cell lines, including HepG2 cells. Wang et al. [2017] proposed that the cytotoxicity of the lipid fractions of oysters could potentially be attributed to apoptosis caused by the increased level of reactive oxygen species in cells and a consequent increase in mitochondrial membrane permeability. Apoptosis in breast cancer BT20 cells and three human pancreatic cancer cell lines induced by EPA was reported by Nappo et al. [2012] and Shirota et al. [2005], respectively. In our study, we observed that the polar lipid fraction had a higher content of EPA compared to the neutral lipid fraction. This observation could potentially explain why the growth inhibition effect of the polar lipid fraction in all three cell lines was higher than that of the neutral lipid fraction. It has been demonstrated that higher n3/n6 ratios play a beneficial role in preventing the development and progression of cancer [Aronson et al., 2001].
CONCLUSIONS
In conclusion, the fatty acid composition of the neutral and polar lipid fractions obtained from C. gigas oysters cultured in Nha Phu Lagoon, Khanh Hoa Coast, Vietnam, exhibited variations throughout the year. The oysters harvested in May and September had a higher content of SFAs in the neutral lipid fraction and a lower content of PUFAs in the polar lipid fraction compared to oysters harvested in January, April, and November. The neutral lipid fraction was predominantly composed of saturated fatty acids, while the polar lipid fraction contained abundant PUFAs. The polar lipid fraction, rich in DHA and EPA, displayed favorable lipid indices, such as the TI and the n3/n6 ratio, which are important in the context of the effects of lipids on human health. The polar lipid fraction extracted from oyster muscle demonstrated stronger inhibitory effects on the growth of the three cancer cell lines (HepG2, MDA-MB-231, and RD) compared to the neutral lipid fraction. This study highlights the potential of C. gigas oysters cultured in Khanh Hoa Coast as a valuable source of PUFA-rich lipids that are beneficial for human health. Although they exhibited weak anticancer activity, they may still hold potential in the prevention and treatment of certain cancer types.