INTRODUCTION
Proper management of meat by-products can unlock significant economic value through appropriate processing and utilization [Alao et al., 2017; Toldrá et al., 2023]. These products include an extensive variety of items, many of which are abundant in vital nutrients like vitamins, minerals, and amino acids [Henchion et al., 2016; Mora et al., 2019; Seong et al., 2014]. The extent to which these by-products are accepted as food varies widely across cultures and geographical areas; what is considered a delicacy in one country might be deemed not edible in another [Alao et al., 2017]. Significantly, organ meats such as liver, heart, kidney and spleen that are rich in nutrients have been adopted into the cuisines and eating habits of different societies all over the world indicating their global importance [Nollet & Toldrá, 2011]. The use of horse by-products is deeply entrenched in Kazakhstan’s culinary and cultural traditions [Atambayeva et al., 2023; Zhumanova et al., 2019], illustrating a meticulous approach to utilizing every aspect of the animal. This tradition highlights both the pragmatism and the deep respect the Kazakh people have for horses, creatures that have been essential to their history and daily lives.
In recent years, microbial safety and quality preservation in meat products have become critical research areas due to heightened consumer demand for safe and high-quality food.
Various studies have explored advanced preservation methods, such as organic acid treatments, high-pressure processing, and ultrasound, to extend shelf-life and reduce microbial contamination [Woldemariam et al., 2019]. Organic acids like citric acid are commonly used for their antimicrobial effects, especially when combined with ultrasound, which enhances microbial inactivation through cavitation [Turhan et al., 2022]. These combined methods are particularly effective in inactivating pathogens and preserving meat quality without compromising the product’s sensory attributes, thus meeting the industry’s stringent safety standards. However, despite these advancements, natural casing materials like those derived from horse intestines present unique challenges in microbial management due to their complex structure and susceptibility to contamination.
Multiple factors can affect the quality and characteristics of natural casings, including the age, breed, and food of the animal, as well as the ambient conditions in which the animal was raised. In order to be suitable for practical use, these casings must possess sufficient strength to withstand the forces associated with filling, stuffing, and processing [Bakker et al., 1999; Ockerman & Hansen, 2000]. Bacteria are naturally present in casings with a typical concentration of 4 to 7 log cfu/g [Bakker et al., 1999; Byun et al., 2001; Hammou et al., 2010; Wijnker et al., 2019] and can also contain viral particles within their structure. The study conducted by Çağlar et al. [2018] detected the presence of Escherichia coli in nine out of the 21 casing samples analyzed. Among these samples, two showed notably high levels of contamination, with 5.77 log cfu/g for E. coli and 5.81 log cfu/g for Staphylococci. Another strain of Salmonella spp. was found in two of the casings. Research has indicated that the count of bacteria present in hog and sheep casings can be significant at the beginning. Bakker et al. [1999] reported overall aerobic counts of 6.3 and 5.9 log cfu/g, as well as halophilic bacteria counts of 4.5 and 5.2 log cfu/g. In contrast, Rebecchi et al. [2015] counted, isolated, and described a number of Staphylococcus, Lactobacillus, Bifidobacterium, Vagococcus, and Clostridium bacteria. More detailed bacteriological investigations, such as those carried out by Fahmy et al. [2021] on 90 natural sheep casings (both local and imported) found that imported casings had greater bacterial levels than the local products. Similarly, initial studies by Byun et al. [2001] revealed large numbers of aerobic bacteria, Enterococci, and coliforms in pork and lamb casings.
Ultrasound technology is vital for the safe processing of intestines and the production of high-quality natural casings, such those used for sausages. Utilizing ultrasound during the cleaning and preparation stages of these casings can significantly decrease the presence of microorganisms. According to research published by Lauteri et al. [2023], the use of ultrasonic cavitation can effectively remove biofilms and other contaminants, significantly lessening the risk of impurity. The microbiological safety of beef products is improved by ultrasonic-assisted procedures in addition to their cleaning effects [de Lima Alves et al., 2018; Morild et al., 2011]. For example, after 30 min of sonication at frequencies of 20 and 40 kHz, Sienkiewicz et al. [2017] showed that Salmonella spp. were completely inactivated in both low and high bacterial populations. Moreover, integrating ultrasound with other sanitation methods, such as hypochlorite, mild heat, pressure, steam, or organic acids, has been shown to significantly increase the antibacterial efficacy in meat processing, as evidenced by studies like those conducted by Arroyo et al. [2011], Diez et al. [2008], and Kang et al. [2018]. The capacity of ultrasound treatment to increase the permeability and uniformity of the casings is another reason why it is highly regarded. This ability has the potential to enhance the overall processing qualities as well as the appearance of the finished product. Not only does this application contribute to the safety of food, but it also enhances the functional features of the casings, which is in line with the expectations of the industry for food products that are of high quality and non-hazardous. Combining ultrasound with other antimicrobial methods results in an increase in the effectiveness of ultrasound as an antibacterial agent. Hence, a number of researchers have investigated the synergistic effects of ultrasound in conjunction with other antimicrobial techniques in order to enhance its capabilities in deactivating microorganisms and enzymes. This has been documented by Nicolau-Lapeña et al. [2019], Silveira et al. [2018], and Singla & Sit [2021]. The utilization of organic acids in combination has proved highly effective in deactivating foodborne pathogens [Diez et al., 2009; Irazoqui et al., 2024]. The combined effect of these therapies greatly enhances microbiological safety by utilizing the mechanical force generated by ultrasound-induced cavitation in conjunction with the antibacterial characteristics of organic acids. Combining these two methods not only helps remove biofilms and other microbiological pollutants more thoroughly, but it also improves the acid’s ability to penetrate the casings, resulting in a more even and efficient decrease in pathogen levels. Research has shown that combining both procedures results in more effective elimination of microorganisms compared to using either strategy individually [Görgüç et al., 2021; Singla & Sit, 2021]. Building upon existing research, studies by Morild et al. [2011] and Lauteri et al. [2023] demonstrated the efficacy of ultrasound in reducing microbial load in meat products. Our study employs similar ultrasound frequencies (35 kHz) but examines the combined effect with citric acid, a relatively less explored synergy in this context. Unlike prior studies that used ultrasound independently, we explored how combining it with citric acid may offer a more potent antimicrobial effect, potentially enhancing both microbial safety and mechanical resilience. The outcome is not only more secure, but also of superior quality shells with enhanced sensory and mechanical characteristics, complying with industry benchmarks for both safety and product uniformity. This combined approach represents a great development in food processing technology since it maximizes meat product functional qualities and safety.
The purpose of this research was to completely analyze the microbiological safety and mechanical integrity of natural horse casings following treatment with ultrasound, citric acid, and a combination of both. This inquiry attempted to establish the usefulness of these strategies, both individually and in combination, in boosting the quality and safety of casings used in sausage manufacture. By examining the individual and combined impacts of ultrasonic and citric acid treatments, this study intended to establish optimal processing settings that ensure the highest standards in product safety, functional performance, and customer acceptability. This technique could potentially set new criteria for industry procedures, enhancing the overall quality and marketability of beef products.
This study focused on short-term storage durations (up to 13 days) to assess the immediate impact of ultrasound and citric acid treatments on microbial safety and mechanical integrity of natural horse casings. The chosen timeframe was designed to capture early-stage microbial reductions, which are critical for initial product stability and quality. Although long-term total plate count (TPC) testing over several months would provide valuable insights into the durability of microbial safety and structural improvements, it was outside the scope of this study due to resource and time constraints. Future research will aim to explore these extended effects, offering a more comprehensive understanding of treatment efficacy over prolonged storage periods.
MATERIALS AND METHODS
Horse intestinecollection
Horse intestines (the transverse and small colons with a diameter of 50 to 120 mm) from horses aged 3–4 years and weighing approximately 300 to 360 kg were randomly collected from various retail markets in the Astana provinces, Kazakhstan. The horses were slaughtered following standard procedures that involved severing major blood vessels, bleeding, skinning, and evisceration. Immediately after slaughter, the horse intestine samples were harvested, cleared of visible fats and connective tissues, and rinsed with tap water to eliminate any residual food and feces. Subsequently, horse intestine (about 100 kg) was segmented into roughly 0.5 m long fragments for treatments involving ultrasound, citric acid, or a combination of both.
Experimental design
Samples were collected and treated with citric acid (CA), ultrasound (US), or a combination of both. Details of the treatment parameters are outlined in Table 1. Post-treatment, the samples were stored in sterile conditions at 4°C for assessment across various durations (1, 5, 9, and 13 days). Key metrics assessed include TPC, content of thiobarbituric acid reactive substances (TBARS), pH, tensile strength, and elongation at break. All studies were performed in the Laboratory of Microorganisms Genetics and Biochemistry at the National Center for Biotechnology (Astana, Kazakhstan) based on the international standards.
Table 1
Process variables for the treatment of natural horse casings.
Procedures for treating horse intestines and their storage
Throughout the ultrasound treatment, a refrigeration unit maintained the water temperature at 20°C, regulated by a temperature control system. The experiment utilized a 10 L ultrasound tank (Sapphire UZV-28 TTC, Sapphire LLC, Moscow, Russia), filled with 8 L of distilled water, operating at a frequency of 35 kHz and a power of 200 W. The full experimental set up is given in Table 1. The treatments were conducted as follows: control (water) – intestines were soaked in distilled water for 5 and 10 min; CA treatment – intestines were soaked in citric acid solutions (0.5% or 1.0%, v/v) for 5 and 10 min; US treatment – intestines were immersed in distilled water and subjected to ultrasound at 35 kHz and 200 W for 5 and 10 min; combined treatment – intestines were first soaked in citric acid solutions (0.5% or 1.0%, v/v) for 5 min and subsequently subjected to ultrasound under the same conditions described for the US treatment.
In all treatments, the solution temperature was maintained at 20°C, and the solution-to-intestine ratio was consistently kept at 8:1 (8 L of solution per 1 kg of intestine) to ensure uniform and effective treatment conditions. After treatment, the samples were briefly washed with tap water for 1 min and then dried using wiper papers. Subsequently, the samples were packed in plastic pouches, approximately 0.5 kg per bag, sealed, and stored at 4°C for 1, 5, 9, and 13 days. Each 500 g portion was treated as an experimental unit.
Determination of total plate count
The TPC in horse intestines was determined following the standard procedure [GOST 10444.15-94] maintained by the Euro-Asian Council for Standardization, Metrology, and Certification (EASC). Initially, 25 g of the intestine samples were mixed with 225 mL of peptone water (1 g/L peptone) and homogenized using an HG-202 laboratory dispersant (HT MachineryCo., Ltd., Tokyo, Japan) for 1 min. Subsequent to homogenization, the samples underwent serial dilution in peptone water (from 1:10 to 1:100, v/v). Then, 1 mL of each diluted sample was spread onto the plates of an aerobic plate count equipment (Test-2, Food Eyes, Beijing, China) and incubated at 37°C for 48 h. After incubation, red colonies visible on the plates were counted as TPC, and the results were recorded in logarithmic colony forming units per g (log cfu/g).
pH measurement
pH measurements were conducted by blending 5 g of each sample with 45 mL of distilled water in a homogenizer (HG-202, HT Machinery Co., Ltd., Tokyo, Japan). The pH levels were then assessed using a pH meter (MP220, Mettler Toledo Intl, Greifensee, Switzerland).
Instrumental color analysis
The surface color of the horse intestines treated with CA solutions, US and a combination of CA (1%) and US for 5 min was quantified using a chroma-meter (CR-300, Konica Minolta, Inc., Marunouchi, Chiyoda-ku, Tokyo, Japan), which was calibrated using a white plate (Y=93.5, X=0.3132, y=0.3198). Five distinct measurements were made at various points on the exterior of the samples. The CIE color space was followed in recording the results, which included values for L* (lightness), a* (redness), and b* (yellowness).
Lipid oxidation measurement
The content of TBARS was measured in the samples following each specific treatment – control (water only), CA treatments, US treatment, and combined CA (1%) and US treatment for 5 min – to determine the levels of lipid oxidation, following the approach published by Kang et al. [2018]. In each test, 5 g of the sample were mixed with 15 mL of distilled water, 50 μL of saturated butylated hydroxyanisole, and 20 mL of a solution containing thiobarbituric acid (0.02 M) and trichloroacetic acid (15%, w/v) in a 1:1 (v/v) ratio (TBA/TCA). The mixture was then mixed at 11,000 rpm for 15 s with an HG-202 homogenizer (HT Machinery Co., Ltd., Tokyo, Japan). The total volume of each homogenized sample was increased to 50 mL with an additional TBA/TCA solution and promptly chilled on ice. The samples were soaked in a 90°C-water bath for 15 min. After heating, the samples were rapidly cooled on ice for 20 min before being centrifuged at 3,000×g for 10 min in a Z-216-M centrifuge (Hermle Labortechnik GmbH, Wehingen, Baden-Württemberg, Germany).
Approximately 1.5 mL of the supernatant was then collected from each sample, and its absorbance was determined at 531 nm using a UV-visible spectrophotometer (UV-VIS-AgilentCary-60, Agilent Technologies Inc., Santa Clara, CA, USA). TBARS levels were calculated based on the weight of the sample and expressed in mg of malondialdehyde per kg of sample (mg MDA/kg). This procedure was repeated three times for each sample in each treatment.
Determination of mechanical properties of the horse intestine casings
The mechanical properties for both the treated casings (with CA solutions, US and a combination of CA (1%) and US) and the control were assessed using a tabletop testing machine (Instron, Model-1026, Norwood, MA, USA)immediately after each treatment session of 5 min. Samples of the casing were prepared by cutting them into strips measuring 120 mm in length and 50 mm in width, corresponding to the width of an open casing, aligned longitudinally. These were then secured within the grips of the equipment, set at an initial distance of 40 mm. Throughout the traction tests, which progressed at a rate of 12 mm/min, data on tension and relative deformation were continuously gathered. Tensile strength (MPa) and elongation at break (ε, %) for each film were determined based on five replicated measurements of the tension/deformation profiles [Okamoto, 1978].
Statistical analysis
The experiment was performed in triplicate, with three independent trials conducted for each treatment condition. After each treatment and at each storage time interval, two samples per trial were analyzed, resulting in a total of six samples per condition per storage time. A mixed-effects model was used to evaluate the effects of citric acid concentration, ultrasound treatment, treatment duration, and storage interval, as well as their interactions, on the response variables: TPC, TBARS, and pH. This model accommodated the complexity of the design, allowing for repeated measures on the same samples over time. Fixed effects included the main factors and their interactions, while random effects accounted for within-sample variability. Analysis of variance (ANOVA) was applied to determine the significance of each main effect and interaction on the response variables. Post-hoc comparisons were conducted using Duncan’s multiple range test at a 0.05 significance level to identify significant differences among treatment combinations. All statistical analyses were performed using SAS software (Version 8.1, SAS Institute Inc., Cary, NC, USA).
RESULTS AND DISCUSSION
Total plate counts and pH
Table 2 outlines the variations in TPC of horse intestine subjected to ultrasound treatment, varying concentrations of citric acid washes, and their combined effects during cold storage. Over time, TPC significantly increased (p<0.05) with longer storage durations. By the final day of storage (day 13), TPC levels in the control samples (A1, A2) were significantly higher (p<0.05) than those in the treated samples, with the exception of some citric acid-only treatments, where no significant differences were observed.This indicates that while citric acid effectively reduced microbial counts initially, different concentrations and durations did not significantly alter its long-term efficacy. The combination of citric acid (1%) with ultrasound treatment (F1) consistently resulted in the lowest microbial counts throughout storage, demonstrating the potential of the combined treatment to provide enhanced microbial control. While all treatments showed statistically significant changes in TPC (log cfu/g) during cold storage at 4°C (p<0.01), treatment F1 (US + CA (1%), 5 min) yielded the most effective control over microbial growth. On day 1, the treatment F1 showed a trend of lower TPC (1.63log cfu/g) compared to the other treatments; however, these differences were not statistically significant (p≥0.05), except in comparison to C1and C2, as indicated in Table 2. Over the storage period, F1 consistently maintained lower microbial counts compared to the other treatments, but these results highlight observed trends rather than definitive differences, emphasizing the importance of analyzing subsequent storage days for more pronounced effects. The observed trend suggests that citric acid concentration was a critical factor in combination with ultrasound in controlling microbial growth. Combination treatment US + CA (1%) was generally more effective than single treatments (either citric acid or ultrasound alone). These insights could guide optimization strategies for food preservation processes, particularly for products susceptible to microbial spoilage. Our findings align with previous studies that highlight the microbial reduction capability of ultrasound at similar frequencies and intensities. For instance, Morild et al. [2011] reported effective reduction in bacterial loads in pork using ultrasound. In turn, Lauteri et al. [2023] found similar effects in beef products. However, our combined ultrasound and citric acid treatment achieved enhanced microbial control compared to ultrasound alone, consistent with recent research by Silveira et al. [2018], who noted the synergistic effects of ultrasound with organic acids on microbial deactivation. This combined treatment approach appears to significantly increase the efficacy of citric acid for pathogen control in casings, presenting a promising sanitization strategy for fresh animal by-products in food processing. Moreover, it is known that citric acid and its salts, in addition to their preservative effect, have a positive effect on the organoleptic properties of natural casings, improve the color and odor of intestines, and an aqueous solution of citric acid is used to remove the unnatural musty odor of casings if it appears during the storage of intestinal raw materials [Sidorova, 2011].
Table 2
Total plate counts (log cfu/g) in horse intestine subjected to various treatments during storage at 4°C.
[i] Results are shown as mean ± standard deviation (n=6). Lowercase letters within each row indicate significant differences across storage time for the same treatment (p<0.05). Capital letters within each column indicate significant differences between treatments for the same storage day (p<0.05). **p<0.01; CA, citric acid; US, ultrasound.
It is advisable to promptly remove internal organs from animals within 30 min post-slaughter to uphold quality standards. According to Galarz et al. [2016], higher temperatures can notably shorten the shelf-life of chicken breasts, varying from 10 to 26 days at 2°C and from 4 to 8 days at 10°C. Furthermore, Hanna et al. [1982] observed changes in bacterial counts in beef, pork, and lamb organs over a 5-day storage period at 2°C. In turn, Kang et al. [2014, 2018] stressed the importance of thorough washing of the small intestine to eliminate blood, indigestible feed, and feces, emphasizing the critical role in reducing bacterial loads to enhance shelf-life and maintain freshness.
Ultrasound treatment applied to fresh intestines resulted in significant changes in TPC over the storage period, but it did not consistently inhibit TPC. The reduction in microorganisms is largely attributed to cavitation, a physical phenomenon described by several authors [Alarcon-Rojo et al., 2019; Bhargava et al., 2021; Zupanc et al., 2019]. Cavitation involves the formation and oscillation of vapor bubbles within a liquid at pressures below its vapor pressure, which grow and implode powerfully enough to detach and deactivate pathogens on submerged surfaces. Our study findings indicated no significant difference in microbial reduction between 5-min and 10-min ultrasound treatments, suggesting the most effective microbial inhibition within the first 5 min, with no substantial increase in effectiveness thereafter. This suggests that ultrasound alone might not suffice for food industry standards due to its limited sanitizing effectiveness and the relatively long treatment times required. Therefore, to develop ultrasound as a viable, short-duration food processing intervention, it should be combined with other methods such as heat, pressure, or organic acids to effectively inactivate and detach foodborne pathogens [Earnshaw et al., 1995; Onyeaka et al., 2021; Silveira et al., 2018]. Our findings are consistent with those of Aguilar et al. [2021], who reported the inactivation of three types of microorganisms using a 10-min ultrasound treatment on raw meat emulsion.
The citric acid treatments (e.g., B1, B2, C1, C2, E1, F1) demonstrated varying degrees of effectiveness in lowering TPC, with significant reductions observed (p<0.01). Reductions in microbial counts were evaluated by comparing each treatment group to the control samples, which were intestines soaked in water. Table 2 provides details on the significant and non-significant differences between treatments at each storage interval. For both concentrations, the 10-min treatments (B2 and C2) showed slightly better control of TPC than the 5-min treatments (B1 and C1). However, the differences between 5-min and 10-min treatments were not substantial. The effectiveness of organic acids in inhibiting microbial growth is influenced by several factors, including pH, the proportion of undissociated acid, the acid chain length, and the cellular physiology and metabolism [Ji et al., 2023]. Lipophilic organic acids, such as sorbic acid, benzoic acid, and lauric acid, can penetrate cell plasma membranes, lowering the internal pH and disrupting cellular function, which contributes to their antimicrobial properties [Ben Braïek & Smaoui, 2021; Sullivan et al., 2020; Ullah et al., 2012]. The reduction in TPC became more pronounced as the pH decreased over time, especially in treatments with higher concentrations of citric acid (from 0.5% to 1.0%), indicating an enhanced antimicrobial effect. However, microbial reduction levels stabilized at a 1.0% CA concentration, consistent with findings from Dan et al. [2017] and Yılmaz Eker et al. [2024].
Table 3 shows the alterations in pH values for horse intestines under various treatment conditions during cold storage. Significant decreases in pH were observed only for the treatments C1, C2, E1, and F1, particularly in those with higher concentration of citric acid (1%) and/or combined ultrasound and citric acid treatment. Other treatments did not produce statistically significant changes over time. This pattern indicates that higher citric acid concentrations and certain treatment combinations were more effective in achieving and maintaining lower pH levels, which contribute to the antimicrobial properties of the treatments. These treatments not only resulted in the lowest pH levels but also demonstrated significant statistical differences, with some showing highly significant reductions (p<0.01). Increasing the concentration and duration of citric acid treatment led to more pronounced pH reductions, as evident from the differences between B1/B2 (CA (0.5%) treatments) and C1/C2 (CA (1%) treatments). The CA (1%) treatments were more effective in lowering pH than the CA (0.5%) treatments. This finding aligns with observations made by Kang et al. [2018], who reported similar pH stability in pig small intestine after seven days of cold storage, highlighting the stringent requirements for thorough washing processes in animal intestines to maintain quality during cold distribution. We can conclude that both combinations of citric acid concentration and ultrasound treatment effectively reduce the pH of horse intestines during cold storage, with the CA (1%) treatment showing a more pronounced and consistent acidification effect. This trend is crucial for the food processing industry, as lower pH levels can help extend the shelf-life of natural casings by inhibiting the growth of spoilage and pathogenic microorganisms. The consistent reduction in pH levels demonstrates that these treatment methods can effectively ensure the safety and quality of sausage casings in commercial settings, providing critical insights for optimizing food preservation processes.
Table 3
pH of horse intestine subjected to various treatments during storage at 4°C.
[i] Results are shown as mean ± standard deviation (n=6). Different lowercase letters within the same row indicate significant differences across storage time for the same treatment (p<0.05). Different capital letters within each column indicate significant differences between treatments for the same storage day (p<0.05). *p<0.05; **p<0.01; ns, not significant (p≥0.05); CA, citric acid; US, ultrasound.
Shelf-life refers to the duration for which a product remains safe for consumption while retaining acceptable sensory qualities. Meat spoilage is primarily driven by microbial activity, oxidative changes, and enzymatic breakdown [Soladoye et al., 2024]. While specific microorganisms are known to cause spoilage [Odeyemi et al., 2020; Zhu et al., 2022], it is often the result of complex interactions among diverse microbial populations. Notably, spoilage can occur even in the absence of microbial activity; for instance, vacuum-sealed sterile meat can deteriorate and develop a bitter taste due to the proteolytic breakdown of meat proteins by endogenous enzymes [Abril et al., 2023]. The progression of meat spoilage is often indicated by changes in odor, which serve as a useful measure for assessing spoilage in various meats, including pork, beef [Djordjević et al., 2019; Yu et al., 2022], and chicken [Katiyo et al., 2020]. These odorous characteristics, ranging from “fresh” to “spoiled”, reflect similar patterns of microbial and chemical changes across different types of meat. Moreover, lowering the pH has been shown to effectively inhibit microbial growth, thereby enhancing the preservation quality of food [Atasoy et al., 2024]. This aligns with our findings that reducing pH, particularly through the use of citric acid in combination with ultrasound, significantly improves the shelf-life of horse intestines during cold storage. In the meat industry, preserving meat and its products is critical. Various techniques such as a gamma irradiation, e-beam irradiation, high-pressure processing, and pulsed electric field have been explored for their bactericidal effects in packaged products, aiming to extend both shelf-life and safety [Amit et al. 2017]. In this study, the combination of citric acid solutions and ultrasound treatment proved to be an effective method for improving both the shelf-life and safety of horse intestines during cold storage.
Color and thiobarbituric acid reactive substances
The results presented in Table 4 reveal changes in the color parameters of horse intestines during cold storage at 4°C following treatments with citric acid, ultrasound, and their combination. The L* values, representing the lightness of the samples, showed significant variation over the storage period and minimal differences between the treated horse intestines. This suggests that the treatments did not noticeably affect the light reflectance properties of the samples, and the perceived lightness remained largely unchanged. The a* values, representing redness, exhibited minimal variation across treatments and no significant changes during storage, indicating stable redness throughout the study. The most noticeable changes were found in the yellowness (b*) values. The observed increase in b* values, which indicates a rise in yellowness, can be attributed to several underlying biochemical processes. One primary factor influencing color changes during storage is lipid oxidation, commonly observed in meat products, which leads to the formation of yellowish pigments, including compounds like aldehydes. Carotenoids, naturally yellow pigments in meat, may also contribute to higher b* values [Amaral et al., 2018]. Furthermore, ultrasound treatment, known for inducing cavitation, can cause protein denaturation, altering the structural characteristics of proteins and affecting light reflectance on the meat surface, thereby influencing color changes, including an increase in yellowness [Roobab et al., 2024]. The influence of pH, particularly in the treatments involving CA (1%), also plays a critical role; the lower pH achieved through these treatments can affect the stability of meat pigments, promoting the development of yellow hues due to the stabilization of oxidation products and altered protein structures [Renerre, 2007]. Overall, the a* values remained stable throughout storage, while the increase in b* values highlights the role of biochemical changes in influencing yellowness, particularly under the effects of lipid oxidation, protein denaturation, and pH modifications from the applied treatments.
Thiobarbituric acid reactive substances serve as a measure of lipid oxidation and are commonly used to assess the extent of secondary lipid oxidation in food products [Abeyrathne et al., 2021]. As depicted in Figure 1, TBARS levels showed variations across the different treatment groups over time, with notable differences emerging as the storage period progressed. Specifically, during storage, the samples treated with both ultrasound and citric acid exhibited significantly lower TBARS values (p<0.05) compared to those subjected to either treatment alone. This suggests that the combination of citric acid and ultrasound was the most effective method for minimizing lipid peroxidation in horse intestines during cold storage. The combined treatment maintained the lowest and most stable TBARS values throughout the storage period, indicating its effectiveness in inhibiting lipid degradation and potentially preserving the quality of the casings better than the treatments using citric acid or ultrasound alone. This outcome is consistent with the broader context of our findings, where the combined effect of citric acid and ultrasound was also observed in maintaining pH stability and reducing microbial load, as seen in Tables 3 and 4. The observed combined effect between citric acid and ultrasound in preventing lipid oxidation could be due to the more uniform and effective penetration of the acid into the tissue, enhanced by the mechanical effects of ultrasound, which may disrupt cell membranes and improve the distribution and action of the citric acid. In the meat processing industry, enhancing the value of lower-priced meat by-products through such technological and scientific advancements is essential. The findings suggest that this combined treatment not only extends the shelf-life but also maintains the overall quality of the product, making it a particularly promising method for refrigerated storage of meat products. Implementing such approaches can significantly increase the economic value of these by-products, thereby benefiting the overall meat production industry [Ahmed et al., 2020; Elgadir & Mariod, 2023; Jayathilakan et al., 2012].
Figure 1
Content of thiobarbituric acid reactive substances (TBARS) in horse intestine subjected to various treatments for 5 min during storage at 4°C. CA, citric acid; MDA, malondialdehyde equivalent; US, ultrasound.
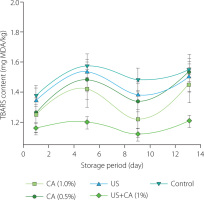
Table 4
Parameters of color of horse intestines subjected to various treatments during storage at 4°C.
[i] Results are shown as mean ± standard deviation (n=6). Different lowercase letters within the same row indicate significant differences across storage time for the same treatment (p<0.05). Different capital letters within each column, separately for each parameter, indicate significant differences between treatments for the same storage day (p<0.05). *p<0.05; **p<0.01; ns, not significant (p≥0.05); CA, citric acid; US, ultrasound; L*, lightness; a*, redness; b*, yellowness.
Mechanical properties
Figure 2 presents the results of traction tests performed on casings subjected to four distinct treatments – CA (0.5%), CA (1%), US alone, and a combination of US with CA (1%) – alongside a control sample. All treatments were administered for a duration of 5 min. This specific time frame was selected based on previous findings indicating that a 5-min combination of acid treatment and ultrasound yielded the optimal outcomes in terms of microbial stability and pH control. The data from these tests are quantified in terms of tensile strength (TS, MPa) and elongation at break (ε, %) [Srinivasa et al., 2007], which reflect the chemical and physical properties of the casings. The results presented in Figure 2a demonstrate that the tensile strength of the casings was significantly influenced by the treatments. The combined treatment (US + CA (1%)) showed the highest tensile strength (5.7 MPa), followed by the 1% citric acid treatment (5.2 MPa), both of which were significantly higher (p<0.05) than the control (4.6 MPa) and other treatments. These findings indicate that the combined treatment and 1% citric acid treatment enhanced the structural integrity of the casings compared to the control and other treatment groups. This improvement can be attributed to the synergistic effects of citric acid and ultrasound, which may have strengthened the collagen fibers within the casings. Moreover, all treated casings showed an increased elongation capacity compared to control, with an average increase of approximately 77% (from ε=28% to ε=45–58%). This increase in flexibility is typical of protein- and carbohydrate-based films that have been plasticized with substances like glycerol or sorbitol [GonzálezTorres et al., 2021]. The intestinal bands of horses, which are part of the outer longitudinal muscular layer of the large intestine and are rich in elastin and collagen fibers, demonstrate the protein-based structure’s susceptibility to plasticizers [Lopes & Pfeiffer, 2000]. Among the treated samples, those subjected to the combined treatment of ultrasound and 1% citric acid exhibited the highest elongation percentage (58%), followed by the 1% citric acid treatment (50%) (Figure 2). The 0.5% citric acid and ultrasound-only treatments also showed moderate improvements in elongation percentages compared to the control but were not as effective as the combined treatment or the 1% citric acid treatment. These results highlight the superior performance of the combined treatment and higher citric acid concentrations in improving the mechanical flexibility of the casings.This treatment could offer significant benefits in applications where enhanced flexibility and structural integrity are critical, such as in the manufacture of natural sausage casings where both properties are essential for optimal performance during filling and handling. The data suggest that integrating ultrasound with citric acid could be a promising method for improving the quality and processing characteristics of natural casings in the food industry. However, to further validate the efficacy and durability of this treatment, additional research is needed to examine how these mechanical properties hold up under different storage conditions and varying environmental factors. Future work will help determine the long-term viability of the ultrasound and citric acid treatment, ensuring that the improvements in flexibility and strength are maintained throughout the product’s shelf-life and under commercial usage scenarios.
Interactions between effects
The results from the ANOVA, detailed in Table 5, highlight the main effects and interaction terms that were statistically significant for various measurements. The significant main effects of citric acid concentration, ultrasound treatment, and storage interval on TPC, TBARS, and pH indicate that these factors individually exert a strong influence on microbial load, lipid oxidation, and the acidity of the casings.
Table 5
Results of analysis of variance (ANOVA) for main effects and interactions.
The significant interaction terms, such as citric acid concentration and ultrasound treatment (C×U), citric acid concentration and storage time (C×S), and ultrasound treatment and storage time (U×S), indicate that the effect of one factor was influenced by the levels of another factor. For example, the interaction between citric acid concentration and ultrasound treatment (C×U) showed that the combined application of these treatments had a more pronounced impact on TPC, TBARS, and pH levels than either treatment alone. Specifically, higher concentrations of citric acid in conjunction with ultrasound treatment result in greater reductions in TPC and TBARS, as well as more stable pH levels over time. Similarly, the interaction between citric acid concentration and storage time (C×S) demonstrates that the effectiveness of citric acid in controlling microbial growth and maintaining pH varies with the duration of storage, indicating that citric acid’s effects are sustained or even enhanced over longer periods. These interactions underscore the importance of combining treatment factors to achieve optimal microbial stability and quality preservation in storage. The extended analysis elucidates the significant impacts of each treatment and their interactions on the quality and preservation of horse intestine. The clear reporting of statistical values, alongside a detailed interpretation of what these effects entail, provides a thorough understanding of how these treatments can be optimized for better food preservation outcomes. This detailed statistical approach ensures that the findings are not only statistically significant but also practically relevant, providing valuable insights for food processing industries.
CONCLUSIONS
The combined application of ultrasound and citric acid has been shown to be more effective in reducing total plate counts than citric acid alone, without compromising product quality. This dual approach enhances microbial safety, reduces acid usage, and shortens processing times, offering cost savings for the meat industry while maintaining food quality. The treatment not only lowered pathogen presence on natural casings but also extended shelf-life and improved mechanical properties. Casings processed with this method were stronger and more elastic, reducing losses and costs in sausage manufacturing. Despite significant changes in yellowness, the combined treatment of 1% citric acid with ultrasound proved to be the most effective method for microbial load reduction and quality preservation of horse intestines during storage. While some degree of color change was expected due to biochemical reactions during treatment, the enhanced microbial safety and extended shelf-life outweigh these minor visual impacts. These findings establish the combined treatment as a promising approach for managing foodborne pathogens and maintaining quality in meat products, especially in applications requiring sustainable and efficient preservation strategies. However, the efficiency of ultrasound, driven by cavitation, can be influenced by factors such as organic matter, water hardness, and dissolved gases, necessitating further trials to optimize conditions for industrial applications. This study highlights the potential for the food processing industry, particularly in sectors emphasizing natural and minimally processed solutions, to adopt ultrasound and citric acid treatments for enhanced microbial safety and optimized processing.
Future research should concentrate on a detailed investigation of the combined ultrasound and citric acid treatment to eliminate foodborne pathogens in inoculated horse intestine casings. While this approach has demonstrated significant potential in enhancing microbial safety, further studies are required to fine-tune the conditions for maximum efficacy. Furthermore, exploring the impact of this combined treatment on the quality properties and shelf stability of sausages made from treated casings will yield valuable insights. Such research should assess the effects on texture, flavor, color, overall consumer acceptance, and how mechanical properties fare under different storage conditions and environmental factors, thereby informing best practices for industrial applications. Examining the long-term stability and microbial resistance of treated casings could lead to significant advancements in food safety and quality, potentially revolutionizing meat processing techniques. The implications of these findings could extend beyond the meat industry, offering novel methods for preserving and enhancing the safety of various perishable food products.