ABBREVIATIONS
GUPP, peel powder of guava; MYPP, peel powder of mamey sapote; PFPP, peel powder of passion fruit; AOAC, Association of Official Analytical Chemists; WHC, water-holding capacity; OHC, oil-holding capacity; GAC, glucose adsorption capacity; DNS, 3,5-dinitrosalicylic acid; FOB, fat/oil binding; SCBC, sodium cholate binding capacity; CAC, cholesterol adsorption capacity, DPPH•, 2,2-diphenyl-1-picryl-hydrazyl radical; ABTS, 2,2’-azino-bis(3-ethylbenzothiazoline-6-sulfonic acid); TSP, total soluble phenolics; TEAC, Trolox equivalent antioxidant capacity.
INTRODUCTION
Guava, mamey sapote, and passion fruit are tropical fruits accessible in international markets; they are consumed fresh, canning, frozen, or as marmalades, nectars, among others. The processing operations of guava, mamey sapote, and passion fruit generate by-products, such as seeds, peels, and unusable pulp, that may reach up to 52% of the fruit weight [Sagar et al., 2018]. These by-products can be reused due to their content of bioactive compounds; among them, dietary fiber which has interesting characteristics like water and oil holding capacity and fat acid adsorption, which increases their excretion and favorably impacts the consumer’s lipid profile [Savran et al., 2016], while the phenolic compounds have shown antioxidant activity besides diverse health-promoting effects, such as acting in the control of postprandial hyperglycemia and overweight [Marquez-Molina et al., 2023]. Obesity is a metabolic disorder resulting from excessive accumulation of body fat, associated with numerous comorbidities like heart disease, stroke, hypertension, and type 2 diabetes mellitus. The complications of people living with diabetes may be partially mitigated by inhibiting α-amylase and α-glucosidase, two enzymes closely related to the digestion of carbohydrates. This strategy counters postprandial hyperglycemia and maintains a stable glycemia [Gutiérrez-Grijalva et al., 2018]. Thus, consuming food products that contain compounds efficient in slowing α-amylase and α-glucosidase activity may benefit consumer health. Various efforts have been made to explore plant-based, effective, inexpensive, and non-harmful inhibitors of α-amylase, α-glucosidase, and pancreatic lipase for this purpose. Plant-based foodstuffs, like by-products of tropical fruits, are known to exhibit minimal side consequences in comparison to synthetic drugs [Azizan et al., 2020]. Previous findings have shown that phenolic compounds from guava leaves and passion fruit by-products exerted an in vitro α-amylase and α-glucosidase inhibition capacity, besides important antioxidant activity [Cao et al., 2021; Liu et al., 2014]. Guava, mamey sapote, and passion fruit extracts have diverse bioactive compounds, suggesting they may exert health-promoting bioactivities.
Therefore, this study aimed to characterize tropical fruit peels from guava, mamey sapote, and passion fruit regarding their in vitro physicochemical, technological, antioxidant, antidiabetic, and antiobesogenic activities. This characterization will allow the proposal of possible applications for the peels instead of discarding them unused, thereby generating additional value for producers, industries, and the consumer.
MATERIALS AND METHODS
Vegetable materials
Guava (Psidium guajava L.), mamey sapote (Pouteria sapota (Jacq.) H.E. Moore & Stearn) and passion fruit (Passiflora edulis f. flavicarpa) in commercial ripeness were acquired in the Central de abasto in Hermosillo, Mexico. Guava, mamey sapote, and passion fruit without apparent damage to the skin and free of molds were chosen, then washed in tap water and disinfected with 0.3 mL/L sodium hypochlorite for 3 min. Clean guavas and passion fruits were cut in half using a sanitized stainless-steel knife, pulp and seeds were removed entirely, and peels of mamey sapote were manually eliminated. The peels were dried in an air circulation oven at 40°C for 24 h. Dry peels were ground in a coffee grinder to obtain flour. Dry peel powders of guava (GUPP), mamey sapote (MYPP), and passion fruit (PFPP) were stored in amber vials at 4oC in the dark until subsequent analysis.
Determination of proximate composition and physicochemical properties
Moisture, proteins, lipids, and ash content determinations were performed according to Association of Official Analytical Chemists (AOAC) methods [Helrich, 1990]. Contents of total dietary fiber (TDF), insoluble dietary fiber (IDF), and soluble dietary fiber (SDF) were established using Asp’s method [Asp et al., 1983]. The peel powders were mixed with distilled water (1:10, w/v), and the pH of the mixture was measured with a pH meter (Fisher Scientific AB150, Ottawa, Canada). Water activity (Aw) was measured at 25°C, utilizing an Aqualab CX 2T apparatus (Decagon Devices Inc., Pullman, WA, USA). Tristimulus color parameters, i.e., lightness (L*), red-green chromaticity coordinate (a*), and yellow-blue chromaticity coordinate (b*), were measured using a CR-400HS colorimeter (Konica Minolta Sensing, Inc., Osaka, Japan). All parameters were analyzed in triplicate.
Determination of techno-functional properties
The water-holding capacity (WHC) and oil-holding capacity (OHC) of fruit peel powders were evaluated according to the procedure proposed by Wu et al. [2010]. In brief, 1 g of peel powder was mixed with 10 mL of water or 10 g of oil in a tube. The mixture was stirred for 1 min, then left to rest for 30 min, and centrifuged at 6,706×g (Allegra 64R centrifuge, Beckman Coulter, La Brea, CA, USA) for 30 min. After this time, the supernatant was carefully poured out, and the pellet with retained water or oil was weighed. The results were recorded as the amount of water adsorbed per g of peel powder (mL water/g) or oil adsorbed per g of peel powder (g oil/g).
Determination of physio-functional characteristics
Glucose adsorption capacity
Glucose adsorption capacity (GAC) of fruit peel powders was evaluated using the procedure described by Ou et al. [2001]. In brief, 0.5 g of peel powders were mixed with 25 mL of a glucose solution at different concentrations (10–200 mM), then incubated at 37°C for 6 h with shaking at 120 rpm. The mixtures were centrifuged at 6,706×g at 25°C for 20 min. After this, 1 mL of the supernatant was carefully poured and then mixed with 0.75 mL of the reagent with 3,5-dinitro salicylic acid (DNS) (0.63% DNS, 0.5% sodium sulfite, 18.5% sodium potassium tartrate, 2.1% sodium hydroxide, and 0.5% phenol) in order to determine the glucose concentration [Fu et al., 2010]. The reaction mixture was incubated at 97°C for 5 min; then left to stand until it reached 25°C; next, 10 mL of distilled water were added. The absorbance of the mixture was determined at 540 nm in a photodiode array spectrophotometer (Model 8452A; Hewlett–Packard Co., Waldbronn, Germany). GAC was reported as mmol of glucose adsorbed per g of peel powder (mmol/g).
Fat/oil binding capacity
Fat/oil binding (FOB) capacity of guava, mamey sapote, and passion fruit peel powders was measured according to the method used previously by López-Marcos et al. [2015]. In brief, 0.2 g of peel powders were weighed in a flask, and 20 mL of 0.16 M HCl was added and shaken at 120 rpm for 1 h. Then, 20 g of oil was added to the flask. The mixture was stirred in a vortex for 1 min before neutralizing it with 0.01 M NaOH. The flask was then kept at 25°C for 24 h, the unbound oil was carefully decanted, and the pellet was recovered by filtration. FOB was reported as the weight of oil adsorbed per g of peel powder.
Sodium cholate binding capacity
Sodium cholate binding capacity (SCBC) of fruit peel powders was measured according to the method described by Xu et al. [2015]. In brief, 100 mL of a solution of sodium cholate (150 mM) in phosphate buffer (pH 7.0) was mixed in a flask with 200 mg of each peel powder and stirred at 120 rpm and at 37°C for 2 h. After this time, the mixture was centrifuged at 760×g for 20 min. The supernatant (0.5 mL) was mixed with H2SO4 (240 μL, 45%, v/v), vortexed, incubated at 65°C for 30 min, and cooled at room temperature, then the absorbance was measured at 620 nm. The concentration of unbound sodium cholate was determined based on a standard curve using a standard of sodium cholate. Results were expressed as mg of bound cholesterol per g of peel powder.
Cholesterol adsorption capacity
Cholesterol adsorption capacity (CAC) was determined according to the method described by Luo et al. [2017]. A solution of fresh egg yolk was prepared in distilled water (1:9, v/v) and stirred at 120 rpm for 5 min. Peel powders (1 g) were mixed with 25 mL of the yolk solution and adjusted to pH 2.0 with 6 M HCl to simulate the pH of the stomach, and the second mixture was prepared under the same conditions described above but adjusted to pH 7.0 with 2 M NaOH to simulate the pH of the intestine. The mixtures were set at 37oC for 2 h with shaking at 120 rpm; after this, they were centrifuged at 4,006×g and 25°C for 20 min. The supernatants (2 mL) were diluted with 10 mL of an acetic acid solution (90%, v/v), adding 0.1 mL of o-phtalaldehyde. The absorbance was measured at 550 nm after 10 min of reaction. CAC values were evaluated based on a standard curve for cholesterol. CAC was shown as mg of cholesterol retained per g of peel powder and was calculated according to the following Equation (1):
where: Cy, concentration of the yolk solution (mg/mL), Cd, cholesterol concentration of the yolk solution after being absorbed by peel powders (mg/mL), Cb, cholesterol concentration of the yolk solution containing no peel powders, V, volume of the yolk solution (mL) and M, weight of peel powder (g).Crude extract preparation
Briefly, 1 g of peel powder was homogenized in 20 mL of a methanol:water (80:20, v/v) mixture and sonicated for 30 min. Samples were then centrifuged at 4,006×g and 4°C for 15 min. Supernatants were collected, and the residues were re-extracted twice with 10 mL of a methanol:water (80:20, v/v) mixture. Supernatants were combined and filtered through Whatman® no. 1 filter paper, and the filtrate was utilized to determine the total soluble phenolic compound content, phenolic composition and antioxidant capacity of fruit peels. For the determination of enzyme inhibition by extracts, the filtrate was subjected to rotary evaporation at 40°C to remove methanol, the residual water was freeze-dried, and the dried extract was redissolved in sodium phosphate buffer (0.1 M, pH 6.9) at the analysis time.
Determination of the content of total soluble phenolics
The content of total soluble phenolics (TSP) was determined by the method of Singleton et al. [1965] with some changes. The extract of peel powder (15 μL) was mixed with 15 μL of 2 M Folin-Ciocalteu reagent and 240 μL of distilled water in a 96 well-microplate and mixed slightly for 3 min, then 30 μL of 2 M Na2CO3 were added; microplates were then allowed to stand without light for 90 min. The absorbance was measured at 765 nm in a microplate reader (FLUOstar Omega, B.M.G. Labtech, Durham, NC, USA). TPS content was expressed as mg of gallic acid equivalent per 100 g of peel powder (mg GAE/100 g).
Determination of individual phenolic compounds
The phenolic compounds of guava, mamey sapote, and passion fruit peel extracts were evaluated by the method of Velderrain-Rodríguez et al. [2018] using an UPLC system (Acquity, Waters Co., Milford, MA, USA) with a photodiode array detector. Separation was performed in an UPLC BEH C18 column (1.7 μm, 3.0×100 mm). The phase solvent was A (0.1%, v/v, acetic acid in water) and B (0.1%, v/v, acetic acid in methanol) delivered at a 0.7 mL/min flow rate. The solvent gradient was initially 91% of A and 9% of B (0–11 min), 9% to 14% of B (11–15 min), and 15% of B (5 min). The phenolic compounds were identified by matching their retention times and absorption spectra with their respective standards and their contents were estimated based on calibration curves. The results were expressed as μg/g of peel powder.
Antioxidant capacity determination
DPPH radical scavenging activity
The 2,2-diphenyl-1-picrylhydrazyl (DPPH) radical scavenging activity of peel powders was evaluated using the method of Brand-Williams et al. [1995]. Peel powder extract (20 μL) and 280 μL of 200 μM DPPH• solution were transferred to a microplate, and the mixture was left in the absence of light at 25°C for 30 min. After this time, the decrease in absorbance was determined at 540 nm in a microplate reader. The Trolox curve from 0.05–1 mmol TE/g was used, and the results were expressed as μmol of Trolox equivalent per 100 g of peel powder (μmol TE/ 100 g).
Trolox equivalent antioxidant capacity
Trolox equivalent antioxidant capacity (TEAC) was determined by the method of Re et al. [1999]. The 2,2’-azino-bis(3-ethylbenzothiazoline-6-sulfonic acid) (ABTS) was dissolved in a 2.45 mM potassium persulfate solution to obtain a 7 mM solution. The mixture was kept in the absence of light for 18 h to generate ABTS•+. The ABTS•+ solution was diluted to reach an absorbance of 0.70±0.02 at 734 nm. The extracts of peel powder or a Trolox standard solution (20 μL) were mixed with 255 μL of the ABTS•+ solution and left at 30°C for 7 min in a microplate. The absorbance was measured at 734 nm in a microplate reader.
TEAC was expressed as μmol of Trolox equivalents per 100 g of peel powder (μmol TE/100 g).
Determination of inhibition of enzymes
α-Amylase inhibition assay
The α-amylase inhibitory activity of peel powder extracts was determined according to the procedure described in Worthington Enzyme Manual [Worthington, 1993]. The substrate (50 μL of a 1% solution of starch in sodium phosphate buffer 0.1 M at pH 6.9), α-amylase (13 U/mL, Sigma-Aldrich, St. Louis, MO, USA) dissolved in the same buffer, and 50 μL of fruit peel extracts (0.25 to 2.50 mg/mL) or acarbose (1 mM) as a control, were incubated at 37°C for 10 min. Then, 1 mL of DNS reagent was added, and the mixture was incubated at 85°C for 15 min. Afterwards, the mixture was moved to an ice bath to cool down at 25°C, and 1 mL of distilled water was added. Finally, 250 μL from the mixture was set in a microplate, and the absorbance was read at 540 nm. The α-amylase inhibitory activity of peel powder extracts was reported in percentage and calculated in the following manner using Equation (2):
where: Ablank is the absorbance of the blank (mixture without peel powder extract) and Aextract is the absorbance of the mixture with peel powder extract.In addition, IC50 defined as the concentration of extract required to inhibit 50% of the enzyme activity was established. The IC50 values were obtained from the regression of the logarithm of the extract concentrations versus α-amylase inhibitory activity (%).
α-Glucosidase inhibition assay
The α-glucosidase inhibition assay was performed as established by Cuevas-Juárez et al. [2014]. Briefly, 50 μL of peel powder extracts (0.25 to 2.00 mg/mL) and 100 μL of α-glucosidase (0.6 U/mL, Sigma-Aldrich) or acarbose (1 mM) as control were left at 37°C for 10 min in a microplate. Afterwards, 50 μL of p-nitrophenyl-α-glucopyranoside (3 mM) was added, and the mixture was left another time in the conditions described above. Enzyme activity was determined at 405 nm in a microplate reader. The α-glucosidase inhibitory activity of peel powder extracts was reported as inhibition percentage and as IC50. Both parameters were defined and calculated analogously to those for α-amylase inhibitory activity as described above.
Pancreatic lipase inhibition assay
Pancreatic lipase inhibitory activity of peel powder extracts was evaluated by the method of Worsztynowicz et al. [2014]. In brief, 20 μL of peel powder extracts (0.25 to 3.50 mg/mL) and 20 μL of a pancreatic lipase solution (1 mg/mL) (100–500 U/mg, Megazyme International, Wicklow, Ireland) were incubated at 37°C for 10 min, then 1.8 mL of a cholate solution in 0.1 M sodium phosphate buffer (1.15 mg/mL) containing gum Arabic (0.55 mg/mL) and 20 μL of p-nitrophenyl palmitate (0.01 M) in isopropanol were added, and the mixture was incubated at 37°C for 10 min. The released p-nitrophenyl was determined at 410 nm using a microplate reader after 10-min incubation at 37°C. The pancreatic lipase inhibitory activity of peel powder extract was calculated according to Equation (2), and the IC50 was determined from these values (as described for α-amylase inhibition assay).
Statistical analysis
Experiments were carried out in triplicate, and results were expressed as mean ± standard deviation (SD). An analysis of variance (ANOVA) and Tukey’s test were performed (p<0.05) to determine statistical differences. Data were analyzed using NCSS 2012 statistical analysis software (NCSS LLC, Kaysville, UT, USA).
RESULTS AND DISCUSSION
Proximate composition and physicochemical properties
The proximate composition of the three peel powders is shown in Table 1. Peel powders had moisture contents from 6.06 to 6.55 g/100 g, which is within the range to prevent the proliferation of microorganisms during storage. GUPP moisture content (6.22 g/100 g) was higher than the values reported by Casarotti et al. [2018] (3.97 g/100 g) for this type of guava by-products. MYPP also showed a higher moisture content (6.06 g/100 g) than the one stated by Solís-Fuentes et al. [2015] (4.42 g/100 g), and PFPP showed moisture content of 6.55 g/100 g which was higher than that reported for passion fruit peels by Selani et al. [2016] (5.85 g/100 g) but lower than that found by Dias et al. [2020] (8.29 g/100 g). The protein content of the tested peel powders ranged from 2.07 to 5.02 g/100 g, while their lipid content varied from 1.20 to 4.25 g/100 g (Table 1). The ash content of GUPP and PFPP in this study (0.83 and 6.62 g/100 g, respectively) was similar to that determined by Casarotti et al. [2018] and Selani et al. [2016] for by-products of guava and passion fruit. Ash content in MYPP (4.11 g/100 g) was higher than the value reported by Solis-Fuentes et al. [2015] in mamey sapote peel (3.89 g/100 g).
Table 1
Proximate composition, dietary fiber content, physicochemical and techno-functional characteristics of guava (GUPP), mamey sapote (MYPP), and passion fruit (PFPP) peel powders.
[i] Results are expressed as mean ± standard deviation (n=3). Means with different letters in rows show significant differences (p<0.05). TDF, total dietary fiber; IDF, insoluble dietary fiber, SDF, soluble dietary fiber; WHC, water-holding capacity; OHC, oil-holding capacity; Aw, water activity; L*, lightness; a*, red-green chromaticity coordinate; b*, yellow-blue chromaticity coordinate.
Table 1 depicts the peel powders TDF, SDF, and IDF content. The researched peel powders had a TDF content from 45.18 to 61.42 g/100 g, and a higher content of IDF than SDF in all samples. Such high IDF content suggests the possibility of important applications of the studied peels in food products due to their various physiological impacts on health. For example, high levels of IDF in the diet have been shown to induce satiety and increase fecal volume, thereby contributing to maintaining or improving digestive health while preventing disorders like constipation and colon cancer [Luciano, 2018]. The observed differences in the proximate composition of peels from tropical fruits could be primarily due to the origin of the fruit, then to the specific cultivar used, fruit maturity, post-harvested practices, as well as the treatment to obtain powders. Other important factors affecting these variables are the agricultural practices and the environmental conditions when they were grown [Kim et al., 2019].
Values of pH showed no significant differences between the samples (Table 1). pH is a significant variable because it can define the type of food matrix where the peels can be added without modifying their technological performance. The present study determined that the pH was acidic (4.00–4.13), implying that the samples could be appropriate for their use in similarly acidic food, such as yogurt [López-Marcos et al., 2015]. Regarding Aw, peel powders showed values of 0.22–0.26 (Table 1), comparable to those previously described for powders of by-products from tropical fruits [Casarotti et al., 2018; Selani et al., 2016]. The low values of pH and Aw of the examined peel powders suggest a low possibility of the growth of microorganisms and the development of non-enzymatic or enzymatic damage, further suggesting the viability of using them as potential food ingredients.
Peel powder color depends on the type of fruit, plant cultivar, and maturity stage when by-products were elaborated into powders. During drying, samples are subjected to high temperatures that promote non-enzymatic and/or enzymatic browning reactions [Deng et al., 2018], causing sample darkening. One of the most significant quality attributes in food is the color; thus, the possible changes due to adding peel powders to a food product should limit their potential application to a maximum value. All peel powders showed high L* values (68.26–90.37), with the highest value in PFPP (Table 1). L* is highly related to the type and concentration of pigments, surface water availability, and water content. For a*, the values were different among the peel powders (Table 1). MYPP had the greatest a*, possibly due to its content of red carotenes [Alia-Tejacal et al., 2005]. The highest b* was measured in PPFP, which was statistically similar (p≥0.05) to GUPP, indicating that these peels have a more notable yellow color because of the color of the passion fruit examined.
Techno-functional properties
WHC is a significant hydration characteristic of dietary fiber, both from a technological and physiological viewpoint, and reflects the capability of a moist material to retain water when it is subjected to compression or centrifugation. It includes water bound, water held, and water trapped within cell-wall associated with the fibers [Nelson, 2001]. WHC of PFPP had the highest (p<0.05) value, followed by MYPP and GUPP, with no significant (p≥0,05) differences (Table 1). WHC of peel powder directly correlates with its SDF content, while samples with a high IDF fraction have a lower WHC [López-Marcos et al., 2015].
WHC data suggests that the peel powders may potentially be used in products requiring hydration, such as baked foods, and viscosity, like yogurts. Comparing our results with literature data, PFPP showed a higher WHC (8.56 mL water/g) than rice bran of two varieties (2.76–3.81 mL water/g), while MYPP and GUPP had a higher WHC than durum wheat of two varieties (2.9–3.76 mL water/g) [Jribi et al., 2019].
OHC reflects the capability of a sample to hold oil; it is an important techno-functional characteristic, particularly for cooked meat products, because holding fat stabilizes the emulsion and preserves the flavor of the product [Sánchez-Zapata et al., 2011]. Peel powder OHC values varied from 2.49 to 4.30 g oil/g (Table 1), which were higher than those previously reported in guava and passion fruit by-products (2.03 and 0.7 g oil/g, respectively) [Martínez et al., 2012]. The variation in the results could be related to the porosity of the peels, which can be modified for drying methods and processing time [Elleuch et al., 2011]. To date, no studies describe the techno-functional properties of mamey sapote peel, suggesting that this by-product has been minimally studied and, therefore, its potential use in edible products has not been yet recognized.
Physio-functional properties
Results in Table 2 show that all powder peel samples adsorbed glucose in a concentration-dependent behavior. The GAC of the peel powders may be partly ascribed to dietary fiber content, which promotes glucose molecule adsorption [Qin et al., 2020]. Marquez-Molina et al. [2023] described that fruit by-products could retain glucose in different concentrations, and the quantity of glucose adsorbed may increase as the concentration in the solution increases. It is also remarkable that GAC of MYPP was higher than that of GUPP and PFPP at all tested glucose concentrations (Table 2), probably due to the higher dietary fiber content and lower moisture content, which contributes to their capacity to adsorb glucose.
Table 2
The glucose adsorption capacity (mmol/g) of peel powders of guava (GUPP), mamey sapote (MYPP), and passion fruit (PFPP) in different concentrations of glucose.
Fat/oil binding (FOB) capacity estimates the peel powders’ ability to retain or adsorb fats into its matrix, mimicking food digestion conditions. The FOB capacity of PFPP was 10.2 g/g, which was higher than that of MYPP (4.22 g/g) and GUPP (1.33 g/g) (Table 3). This physio-functional property seems to be related to the SDF/IDF ratio, where higher ratios were associated with higher FOB capacity. FOB capacity of the peel powders analyzed was lower than that reported by López-Marcos et al. [2015] for other fruit by-products like these of grapefruit (35.43 g/g) and pomegranate (14.00 g/g).
Table 3
In vitro fat/oil binding (FOB) capacity, sodium cholate binding capacity and cholesterol adsorption capacity of peel powders of guava (GUPP), mamey sapote (MYPP), and passion fruit (PFPP).
Dietary fiber can bind bile acids in the small intestine and cholesterol in both the stomach and small intestine. Therefore, the sodium cholate and cholesterol binding capacities of dietary fiber-rich materials are important parameters to evaluate [Mudgil & Barak, 2013]. The highest binding capacities was determined in MYPP (2.39 mg/g), followed by GUPP (1.94 mg/g), and PFPP (1.77 mg/g). These different bile-acid-binding values may result from the different TDF content of peel powders.
The cholesterol adsorption capacity of PFPP was significantly higher than those of GUPP and MYPP. The pH value significantly (p<0.05) affected the cholesterol adsorption abilities of all samples, where the CAC values at pH 2.0 were lower than those at pH 7.0. These results indicate that PFPPs have the potential to reduce cholesterol concentration, which could be related to their SDF content [Zhang et al., 2023]. Dietary fiber could disturb the absorption of cholesterol and bile acids in the digestive system, promoting bile acid synthesis from cholesterol, which could decrease the total cholesterol concentration [Niu et al., 2013].
Total soluble phenolic content and phenolic compound profile
The TSP content of fruit peel powders ranged from 185.2 to 365.7 mg GAE/100 g and decreased in the following order: MYPP > GUPP > PFPP (Table 4). TSP values were higher than those described by Can-Cauich et al. [2017] for mamey sapote peel (210 mg GAE/100 g) and guava and passion fruit by-products (254.7 and 175.6 mg GAE/100 g, respectively) [Selani et al., 2016]. The variations among studies can be related to the varieties analyzed, fruit ripening state, drying method, and extraction methods used to process the samples.
Table 4
Contents of total soluble phenolics (mg GAE/100 g) and individual phenolic compounds (μg/g) of guava (GUPP), mamey sapote (MYPP), and passion fruit (PFPP) peel powders.
The individual phenolics determined in the fruit peel powders are listed in Table 4. Gallic, chlorogenic, ferulic, p-coumaric, sinapic, and cinnamic acids, besides catechin and quercetin 3-β-d-glucoside were identified. The identification reported herein agrees with these reported by Cao et al. [2021], who describe the presence of 31 phenolic compounds, including gallic and ferulic acid, catechin and quercetin in passion fruit peel. Liu et al. [2018] determined 69 phenolics in guava peels, among them gallic acid, ferulic acid, catechin and quercetin which were reported in this study, while Yahia et al. [2011] detected gallic and p-coumaric acids, shown in this study, besides syringic and p-hydroxybenzoic acids, hydroxybenzoic acid derivative, p-hydroxybenzoic acid dimer and epicatechin dimer. In turn, Torres-Rodríguez et al. [2011] found gallic acid, gallocatechin-3-gallate and epicatechin in extracts of mamey sapote pulp. According to our literature review, the profile of phenolic compounds in mamey sapote peels has not been reported so far.
Antioxidant capacity
The antioxidant systems chosen for this study were capable of evaluating the activity of hydrophilic compounds (TEAC) and hydrophilic and lipophilic compounds (DPPH assay) [Rufino et al., 2010]. Figure 1 illustrates the antioxidant capacity of peel powders, which varied from 125.3 to 252.4 μmol TE/100 g for DPPH• scavenging activity in the order of MYPP > PFPP > GUPP. Other authors have reported different values of the antioxidant activity of tropical fruit by-products; for example, Casarotti et al. [2018] reported higher DPPH• scavenging activity than those of the present study, at 1,737.11 and 1,340.23 μmol TE/100 g for guava and passion fruit by-products, respectively. However, Martínez et al. [2012] found values like those reported here, with 150 and 330 μmol TE/100 g for guava and passion fruit by-products, respectively. Can-Cauich et al. [2017] reported antiradical activity against DPPH• of 600 μmol TE/100 g of mamey sapote peels, which was higher than those reported herein. Regarding the TEAC, MYPP showed the highest activity (656.8 μmol TE/100 g) (Figure 1). GUPP and PFPP did not differ significantly in this respect (p≥0.05), Martínez et al. [2012] described TEAC of 190 and 210 μmol TE/100 g for guava and passion fruit by-products, respectively, while Can-Cauich et al. [2017] obtained 463 μmol TE/100 g for mamey sapote peel. Our results indicate that mamey sapote peel powder had the highest antioxidant capacity, possibly due to the highest phenolic compound content. Differences in radical scavenging activities are also related to the phenolic compound specific composition of peel powders [Velderrain-Rodríguez et al., 2018].
Figure 1
DPPH radical scavenging activity (A) and Trolox equivalent antioxidant capacity, TEAC (B) of guava (GUPP), mamey sapote (MYPP), and passion fruit (PFPP) peel powders. Values are presented as mean and standard deviation (n=3). Different letters above bars indicate significant differences (p<0.05).
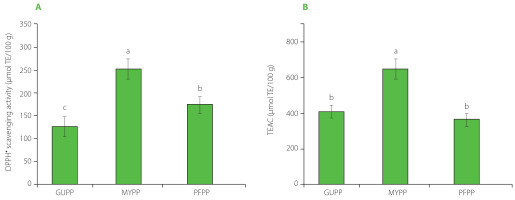
Inhibition of enzymes
The inhibition of α-amylase and α-glucosidase by peel extracts showed an extract concentration-dependent response (Figure 2A, 2B). Both enzyme inhibitory activity of extracts decreased in the order of MYPP > PFPP > GUPP, with a stronger effect against α-glucosidase than α-amylase. The α-amylase and α-glucosidase inhibitory activities of MYPP extract were 42.46 and 64.15%, respectively, at the maximum concentration tested. Suleria et al. [2020] suggest that the presence of ferulic and gallic acid identified in by-products of fruits can inhibit these enzymes. Other studies have confirmed the inhibitory activity of isolated ferulic acid against α-amylase and α-glucosidase and chlorogenic acid against α-amylase [Zheng et al., 2020]. All of these phenolic acids were detected in MYPP, GUPP, and PFPP (Table 4) and may be responsible, at least in part, for the documented effects.
Figure 2
α-Amylase (A) α-glucosidase (B) and pancreatic lipase (C) inhibitory activity of extracts of guava (GUPP), mamey sapote (MYPP), and passion fruit (PFPP) peel powders. Values are presented as mean and standard deviation (n=3). Different letters above bars separately for each extract indicate significant differences (p<0.05).
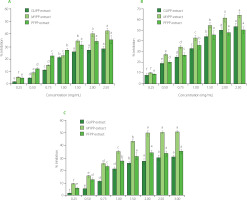
IC50 values against α-amylase were >2.50 mg/mL for all extracts. These data were inferior to the data reported by Liu et al. [2014] for guava leaves (50.5 μg/mL) and to passion fruit by-products (1.8 mg/mL) as reported by Cao et al. [2021]. On the other hand, IC50 values against α-glucosidase were 1.11, 1.24, and 1.57 mg/mL for MYPP, GUPP, and PFPP extracts, respectively, which were inferior to the values reported by Cao et al. [2021] for passion fruit by-products (0.1 mg/mL) and by Liu et al. [2014] for guava leaves (34.6 μg/mL). To date, studies on the inhibitory activity of mamey sapote or its peel against α-amylase or α-glucosidase have not been described.
Even though the inhibitory effects of extracts were lower compared to acarbose (78.22% for α-amylase and 86.42% for α-glucosidase), the data suggest that the peel powders could be good inhibitors. Previous studies have established that fruit by-products can inhibit α-amylase and α-glucosidase with minimum effects [Agada et al., 2020]. Differences in enzyme-inhibiting effects between the peel fruit extracts may be due to the variety of fruits used, their specific phenolic profile, and the enzymes’ origin.
Pancreatic lipase is the enzyme in control of the hydrolysis of fats into monoacylglycerides and free fatty acids in the intestinal lumen [Zdunić et al., 2020]; its inhibition may have beneficial effects on weight loss in diabetic patients. The studied peel powder extracts displayed a dose-dependent lipase inhibitory capacity, presenting an IC50 of 2.61mg/mL for MYPP extract, and >3.50 mg/mL for PFPP and GUPP extracts. The pancreatic lipase inhibitory activity at the highest concentration of extracts (3.5 mg/mL) was 52.93, 34.92, and 30.15% for MYPP, PFPP, and GUPP, respectively (Figure 2C). Gallic and ferulic acid identified in the three peel powders (Table 4) have been reported as pancreatic lipase inhibitors and could be responsible for the observed effect [Ray et al., 2014].
Phenolic compounds in the diet have been demonstrated to be effective inhibitors of the digestive enzymes: α-amylase, α-glucosidase, and pancreatic lipase, which is beneficial for diabetic patients or related conditions [Tan et al., 2017]. Some common clinical management strategies involve the treatment with enzyme inhibitors, like acarbose and orlistat, for α-amylase and α-glucosidase and pancreatic lipase [Gutiérrez-Grijalva et al., 2019]. However, constant consumption of these drugs causes adverse effects related to gastrointestinal pain and oily spotting. Thus, natural alternatives like phenolic compounds from tropical fruits are currently being studied since they can have fewer or no secondary effects.
CONCLUSIONS
The guava, mamey sapote, and passion fruit peel powders showed high TSP content and good antioxidant capacity measured as the DPPH radical and ABTS radical cation scavenging activity. Their extracts also exerted medium α-amylase and lipase inhibition potential and a stronger α-glucosidase inhibitory activity. The excess generation of free radical remains pivotal in the evolution and problems of obesity and diabetes; hence, guava, mamey sapote, and passion fruit peel powders dual-acting antidiabetic and antioxidant capacities suggest they may be suitable for glycemic and overweight management by putting down carbohydrate and lipid digestion. This research proposes that the mamey sapote, guava, and passion fruit peel powders can be applied as functional components according to their technological and physiological characteristics, making them possible candidates to be utilized as ingredients of innovative food products. Depending on these properties, they can be used to improve texture, reduce sugar and calories in foods, such as bakery and meat products. Further, in vivo investigations on tropical fruit peel powders’ antioxidant and hypoglycemic activities and their potential mechanism of action are necessary.