ABBREVIATIONS
ASP, acerola seed powder; D, diameter; DPPH•, 2,2-diphenyl-1-picrylhydrazyl radical; DW, dry weight; FRAP, ferric reducing antioxidant power; GAE, gallic acid equivalent; IDF, insoluble dietary fiber; OHC, oil holding capacity; SDF, soluble dietary fiber; T, thickness; TDF, total dietary fiber; TE, Trolox equivalent; WHC, water holding capacity.
INTRODUCTION
Acerola (Malpighia emarginata) also known as Barbados cherry or West Indian cherry belongs to Malpighiaceae family, which has recently attracted the attention of consumers thanks to its high ascorbic acid content in fruits [Poletto et al., 2021]. The global acerola extract market is anticipated to reach 17.5 billion dollars by 2026 due to the high demand for natural bioactive-rich fruits and derivatives [Belwal et al., 2018]. The processing of acerola fruit generates considerable amounts of by-products which account for 30% of seeds and peels, and 10% of sludge [Poletto et al., 2021]. Developing new methods that add value to these by-products to minimize the cost of waste management and promote their valorization is therefore essential.
Acerola seeds contain high levels of insoluble dietary fiber (IDF) (75.66 g/100 g dry weight, DW), soluble dietary fiber (SDF) (4.76 g/100 g DW), phenolic compounds (4.73 g/100 g DW) and even ascorbic acid (457.32 mg/100 g DW) [Marques et al., 2013]. Consequently, acerola seeds can be considered as a supplement, rich in both dietary fiber and phenolic compounds, to develop new food products with various health benefits. Dietary fiber has significant implications for gastrointestinal disorders [Gill et al., 2021] and elicits other various benefits to human health, such as supporting the treatment of eating disorders, diabetes and obesity [Basu et al., 2021]. Moreover, products rich in phenolic compounds and dietary fiber exert preventive effects against cardiovascular diseases, obesity, type 2 diabetes and cancers [Khan et al., 2022]. It was reported that acerola seed flour was added to the recipe of cereal bars to enhance their dietary fiber and phenolic contents [Marques et al., 2015].
SDF and IDF serve different physicochemical and physiological functions. IDF improves the movement of material through the gastrointestinal tract facilitating laxation, while SDF supports the growth of gut microbiota and plays an essential role in attenuating the glycemic reaction and plasma cholesterol level [Gill et al., 2021]. The IDF to SDF ratio of a food product provides important information on its nutritional and physiological effects on the consumers. According to the American Dietetic Association, the IDF to SDF ratio should be 3:1 to ensure the maximum health benefits of dietary fiber [Borderías et al., 2005]. Nevertheless, the IDF to SDF ratio of the cereal bars enriched with acerola seed flour was very high and varied from 8:1 to 17:1 [Marques et al., 2015].
Many techniques, such as mechanical degradation, chemical and biochemical treatment, and microbiological fermentation of food by-products, were applied to reduce the IDF to SDF ratio by partial conversion of IDF to SDF [Gan et al., 2021]. Among these methods, enzymatic treatment has attracted considerable attention due to a reduced loss in bioactive compounds and environmental friendliness [Ta et al., 2023]. Deoiled rice bran processed via cellulase treatment improved the quality of dietary fiber attributed to the increase in SDF and a decrease in the IDF to SDF ratio [Cao et al., 2022]. Moreover, cookies incorporated with the cellulase-treated deoiled rice bran, wheat bran, or spent tea leaves were found to have an improved soluble dietary fiber content, a decreased hardness and an increased overall acceptability [Cao et al., 2022; Nguyen et al., 2021; Nguyen et al., 2022].
To the best of our knowledge, the cellulolytic treatment of acerola seeds has not been reported in the literature. In this study, ASP was treated by cellulase at different conditions to investigate the effects of this treatment on its fiber profile and evaluate the quality of cookies fortified with the cellulase-treated ASP.
MATERIALS AND METHODS
Materials and reagents
Acerola (Malpighia emarginata) fruits originated from a local farm in Tien giang province (Vietnam). At the laboratory, the fruits were selected, washed with potable water, blanched at 90°C for 2 min and manually pulped to collect acerola seeds. The seeds were subsequently dried at 50°C for 7–8 h to the moisture content of about 13 g/100 g. The dried seeds were ground, and the obtained acerola seed powder (ASP) was preserved in plastic bags at room temperature for further experimentation. Wheat flour originated from Binh dong Flour Co. (Ho Chi Minh City, Vietnam).
CelluclastⓇ 1.5L with endo-1,4-β-glucanase activity (optimal temperature and pH – 50°C and 4.5–6.0, respectively; catalytic activity – 600 endoglucanase units (EGU)/mL) was purchased from Novozymes A/S (Bagsværd, Denmark). Chemicals of the analytical grade were bought from Aldrich-Sigma (Saint Louis, MO, USA).
Enzymatic treatment of acerola seed powder
The enzymatic treatment of ASP with CelluclastⓇ 1.5L was carried out in 500-mL Erlenmeyer flasks. About 50 g of ASP were placed in each flask. The cellulase preparation was diluted in different volumes of tri-sodium citrate buffer (pH 5.0) and subsequently added into the flasks to achieve the required moisture content of ASP and endoglucanase activity. In the first series, the initial moisture contents of ASP were adjusted to 2, 4, 6, 8, and 10 g water/g DW of seeds, while the cellulase dose and incubation time were fixed at 10 U/g DW of seeds and 60 min, respectively. In the second series, the initial enzyme doses were 0, 2.5, 5, 7.5, 10, and 12.5 U/g DW of seeds, while the treatment was conducted at the initial moisture content of 6 g water/g DW of seeds for 1 h. In the third series, the treatment time was 0, 30, 60, 90, and 120 min, while the initial moisture content and cellulase dose were 6 g water/g DW of seeds and 10 U/g DW of seeds, respectively. The reactions were performed at 50°C in an incubator (Memmert, Schwabach, Germany). The heat treatment by using a water bath at 95°C for 10 min was used to inactivate the enzyme. The obtained products were dried at 60°C in a convection oven until the final moisture content of 10 g/100 g was reached. Dried cellulase-treated ASP was sieved through a 40-mesh screen and stored at 4°C. The chemical composition, antioxidant activity, water holding capacity (WHC) and oil holding capacity (OHC) of the untreated ASP and cellulase-treated ASP were determined under following conditions: initial moisture content of 6 g/g DW, enzyme dose of 10 U/g DW, and treatment time of 90 min. Both ASP types were also used in cookie recipe.
Cookie production at a laboratory scale
The cookie formula used in this study included 200 g flour blend or wheat flour, 70 g isomalt, 70 g raw whole egg, 70 g shortening, 2.4 g sodium bicarbonate, 1.4 g lecithin, 1.0 g table salt, 0.18 g acesulfame potassium, 0.6 g vanilla extract, and 13.0 g water. Flour blends were prepared from wheat flour partially replaced (10, 15, 20, 25 and 30%, w/w) by the untreated or cellulase-treated ASP. Cookies were coded as C (control cookie produced without ASP); AS10, AS15, AS20, AS25 and AS30 (cookies prepared using flour blends with 10, 15, 20, 25 and 30% (w/w) untreated ASP, respectively); CAS10, CAS15, CAS20, CAS25 and CAS30 (cookies from flour blends with 10, 15, 20, 25 and 30% (w/w) cellulase-treated ASP, respectively).
The cookie production was conducted as previously described [Cao et al., 2022]. The cream was performed using a mixer (Model M8, Vietnam Unie Co., Ltd, Vietnam). Whole eggs were whipped at 200 rpm for 4 min. Isomalt and the solution of table salt and acesulfame potassium were incorporated into the whipped egg. The mixture was whipped at 200 rpm for 4 min and creamed with lecithin and butter (200 rpm, 3 min). Vanilla extract and sodium bicarbonate were added to the cream (200 rpm, 3 min). The dough was formed by mixing the final cream with wheat flour or wheat flour with ASP (100 rpm, 2 min). Cookies with a thickness of 4 mm and a diameter of 35 mm were formed by a rolling pin and circular mold, and then baked in a reel oven (VH509S, Sanaky Co., Ho Chi Minh City, Vietnam) at 175°C for 10 min and flowed at 150°C for 9 min. The baked cookies were cooled to room temperature for 30 min and sealed in transparent low-density polyethylene (LDPE) zip bags (Saigon Indochina Co., Ho Chi Minh City, Vietnam). Afterwards, they were kept at room temperature for a minimum of 24 h prior to further analysis.
Determination of chemical composition
The moisture content of wheat flour, ASP and cookies was measured by drying to constant weight at 105°C using a moisture analyzer (A&D Co., Tokyo, Japan). The protein content of wheat flour, ASP and cookies was analyzed using the AOAC International 979.09 method (Kjeldahl method) [AOAC, 2000]. Lipid content was determined by Soxhlet extraction with diethyl ether solvent according to the AOAC International 920.39 method [AOAC, 2000]. Ash content was quantified by incineration at 600°C in a muffle furnace (Hope Valley, Lenton Co., UK) using AOAC International 942.05 method [AOAC, 2000]. Total carbohydrate content was determined by the phenol–sulfuric acid method [Nielsen, 2010]. Starch was quantified using the starch digestion method previously described by Landhäusser et al. [2018]. Starch was broken down using α-amylase (A4551, Sigma Aldrich, Saint Louis, MO, USA) at 85°C for 2 h, and the solids were separated by a centrifuge at 13,000×g for 1 min; the supernatant was hydrolyzed into glucose using amyloglucosidase (Sigma Aldrich, A7095) at 55°C for 2 h, and the resulting glucose in the hydrolysate was quantified using an assay with 3,5-dinitrosalicylic acid (DNS) [Miller, 1959]. Total dietary fiber (TDF), IDF and SDF contents were analyzed following the AOAC International 991.43 method [AOAC, 2010]. Briefly, starch and protein were solubilized and hydrolyzed using α-amylase, protease, and amyloglucosidase. For the measurement of IDF, the digested sample was filtered, and the IDF was determined after subtracting ash and protein weight in the residue. To measure SDF, ethanol 95% (v/v) was added to the filtrate at 60°C with a ratio of 1:4 (v/v) to precipitate soluble fibers. The precipitate was collected by filtration. The SDF was determined after correcting its ash and protein in the precipitate. The sum of IDF and SDF contents represents TDF content. The results of the above mentioned analyses were expressed as g/100 g DW of wheat flour, ASP or cookies.
The phytate content of wheat flour, ASP and cookies was determined by spectrophotometric method formerly outlined by Sureshkumar et al. [2015]. About 1 g of the sample was extracted with 20 mL of 0.5 M HNO3. The filtrate was adjusted to an appropriate volume with distilled water. Then, 1.4 mL of this solution was boiled with 1 mL of a ferric ammonium sulfate solution (21.6 mg/100 mL distilled water) for 20 min. The mixture was cooled and mixed with 5 mL of isopentanol, followed by adding and shaking with 0.1 mL of ammonia solution. The alcoholic layer was separated by centrifugation (500×g, 10 min) to determine the color intensity at 465 nm. Alcohol was used as the blank, and sodium phytate was used as the standard. Ascorbic acid content was measured by the spectrophotometric method previously reported by Bajaj & Kaur [1981]. Results of both analyses were expressed as mg/100 g DW of wheat flour, ASP or cookies.
Determination of total phenolic content and antioxidant capacity
About 1 g of ASP, wheat flour or cookies was extracted with 10 mL of an aqueous acetone solution (60%, v/v) at room temperature for 30 min. The mixture was then centrifuged at 1,600×g for 20 min (3K30, Sigma Zentrifugen Ltd., Osterodeam Harz, Germany). The volume of the supernatant was adjusted to 100 mL with a solvent, and the extract was stored at –4°C in the dark. It was used within 2 days for the determination of total phenolic content and antioxidant capacity.
The total phenolic content was determined by a spectrophotometric method developed by Singleton & Rossi [1965] using Folin–Ciocalteu reagent and the result was expressed as mg gallic acid equivalent (GAE)/g DW.
The 2,2-diphenyl-1-picrylhydrazyl (DPPH) radical scavenging activity was measured using a previously published method [Brand-Williams et al., 1995]. The diluted extract (0.1 mL) was mixed with 3.9 mL of 60 μM methanol DPPH solution. The absorbance at 515 nm was measured at 0 and after 30 min of incubation against methanol as a blank. Iron (III) reducing antioxidant power (FRAP) assay was carried out using the method of Benzie & Strain [1999] with some modifications. The reagent solution was prepared by mixing 25 mL of 300 mM acetate buffer (pH 3.6), 10 mM 2,4,6-tris(2-pyridyl)-s-triazine solution in 40 mM HCl (2.5 mL), and 20 mM FeCl3×6H2O (2.5 mL). The diluted extract (0.2 mL) was mixed with 3.8 mL of the FRAP reagent solution and incubated in the dark at 37°C for 5 min. The absorbance was measured at 593 nm against a reagent blank. The results were expressed as μmol Trolox equivalent (TE)/g DW.
Determination of physical properties
WHC and OHC of untreated, cellulase-treated ASP and wheat flour were measured following AACC approved methods of analysis (56-30.01 method) [AACC, 1983] and the method described by Nguyen et al. [2022], respectively. Briefly, to determine WHC, 3 g of the sample were mixed with 30 mL of distilled water. After 24 h at room temperature, the mixture was centrifuged (1,000×g for 20 min at 20°C). The supernatant was removed to determine the weight of the hydrated material. WHC was expressed as g H2O/g DW. Similarly, OHC was determined in the same procedure in which distilled water was replaced by soybean oil. OHC was expressed as g oil/g DW.
The thickness and diameter of cookies were measured by the method described by Park et al. [2015] using a micrometer. Thickness (T) was calculated by the average thickness of the stack of six cookies. Diameter (D) was determined by the average width of six cookies put edge to edge. The spread factor (D/T) was the quotient of diameter and thickness.
Hardness and fracturability of cookies were explored by a three-point break test with a test speed of 1.0 mm/s, distance between two beams of 20 mm, trigger force auto of 50 g, with a 5 kg loading cell and 5 readings taken with TA-XT plus texture analyzer (Stable Micro Systems, Haslemere, UK).
Color of wheat flour and ASP, and surface color of cookies was measured by Konica Minolta CR400 Chromameter (Osaka, Japan) in CIELAB space. The color parameters included lightness (L*), redness/greenness (a*) and yellowness/blueness (b*). The total color difference (ΔE) was calculated according to the formula (1):
where: L*0, a*0, b*0 are the color values of the control cookie; L*, a*, b* are the color values of the cookie prepared using flour blends.
Determination of overall acceptability
The test of overall acceptability of cookies was carried out with 60 participants (both men and women from 18 to 50 years old) recruited among students and staff of the Ho Chi Minh City University of Technology (Ho Chi Minh City, Vietnam). Each cookie samples were coded by a random three-digit number and served randomly. The overall acceptability of cookies was rated on a nine-point hedonic scale ranging from 1 (extremely dislike) to 9 (extremely like) [Nguyen et al., 2021].
Statistical analysis
Each type of cookies was prepared at least three times and all experimental results were expressed as mean ± standard deviation. One-way analysis of variance (ANOVA) was applied to determine the differences between means using Minitab 16 software (Minitab Co., State College, PA, US). Differences were considered statistically significant at p<0.05.
RESULTS AND DISCUSSION
Effects of cellulolytic reaction conditions on fiber contents of acerola seed powder
The significant alteration was found in dietary fiber profile of the ASP treated with cellulose under different conditions including initial moisture content, cellulose preparation dose, and reaction time (Figure 1). Increasing initial moisture content from 2 to 6 g water/g DW of seeds remarkably increased the SDF content from 3.9 to 7.4 g/100 g DW, but the SDF content was reduced when the initial moisture content further increased (Figure 1A–C). Meanwhile, the IDF content gradually decreased from 57.2 to 51.1 g/100 g DW. Hence, the lowest IDF to SDF ratio was found at 6 g water/g DW of acerola seeds. Regarding the effects of enzyme dose on ASP dietary fiber content, the SDF content was significantly improved by 185% as the enzyme dose increased from 0 to 10 U/g DW of seeds, then slightly dropped to 6.2 g/100 g DW at 12.5 U/g DW (Figure 1D–F). As expected, the lowest IDF to SDF ratio was achieved at an enzyme dose of 10 U/g DW. In addition, the SDF content was elevated by 204% while the IDF content was decreased by 15% after 90 min of cellulolysis (Figure 1G–I). The prolonged incubation time to 120 min led to a drop in both SDF and IDF contents. As a result, the IDF to SDF ratio reached its minimum value of 6.5 at 90 min of enzymatic reaction, however this value did not differ significantly (p≥0.05) from the ratios determined at incubation times of 60 and 120 min.
Figure 1
Content of insoluble dietary fiber (IDF), soluble dietary fiber (SDF) and ratio of IDF to SDF of acerola seed powder treated with cellulase at different initial moisture contents and a constant cellulase dose of 10 U/g dry weight (DW) for 60 min (A–C, respectively), different enzyme doses and a constant initial moisture content of 6 g water/g DW for 60 min (D–F, respectively), and different reaction times and an initial moisture content of 6 g water/g DW and an enzyme dose of 10 U/g DW (G–I, respectively). Different letters (a-e) above bars indicate significant differences (p<0.05).
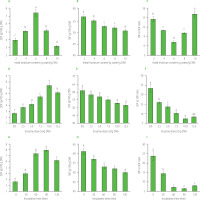
The changes in the ASP dietary fiber profile with respect to the initial water content were in agreement with our previous studies, in which wheat bran was treated with a cellulase preparation at different conditions and the increased moisture content from 0.25 to 0.75 g water/g DM enhancing the content of SDF and decreasing IDF to SDF ratio [Nguyen et al., 2021]. Moisture plays an important role in hydrolytic yield since it probably affects cellulase diffusion and absorption on the cellulose surface which is a prerequisite step in cellulolysis mechanism [Wang et al., 2011]. In the enzymatic treatment of deoiled rice bran, as the cellulase dose increased from 0 to 2 U/g DM, the IDF content decreased by 5% while the SDF content increased by 26% [Cao et al., 2022]. The higher cellulase doses up to 20 U/g resulted in a noticeable transformation of IDF to SDF in the enzymatic treatment of spent green tea leaves [Nguyen et al., 2022]. Generally, doses of cellulase causing a significant effect on the dietary fiber profile in the current study did not agree with those in the previous studies, because of the different origins of cellulosic materials. However, the SDF content of ASP was significantly reduced in all three set experiments at the high initial moisture content (8–10 g water/g DW), excess cellulase dose (12.5 U/g DW), and elongating incubation time (120 min). This phenomenon could be attributed to the intense hydrolysis of dietary fiber into low molecular-weight carbohydrates such as short-chain oligosaccharides and sugars [Bera et al., 2015]. It should be noted that the carbohydrates with the degrees of polymerization 3–9 scarcely precipitate in 78% ethanol solution contributing to the loss in SDF content [Dai & Chau, 2017].
Physicochemical properties and antioxidant capacity of untreated and cellulase-treated acerola seed powder
The cellulolytic reaction conditions at the initial moisture content of 6 g/g DW with an enzyme dose of 10 U/g DW for 90 min were applied for the preparation of the cellulase-treated ASP, whose the chemical composition, antioxidant capacity and physical properties are shown in Table 1. The results achieved for the untreated ASP and wheat flour were also presented (Table 1). The cellulase-treated ASP had a 207% higher SDF content and 73% lower IDF to SDF ratio than the untreated counterpart, whereas the protein, lipid, and starch contents changed insignificantly (p≥0.05) after the treatment. Both cellulase-treated and untreated ASP contained a significantly (p<0.05) higher amount of IDF, SDF, TDF, and ash, and lower amount of starch and protein than wheat flour. Moreover, phytate content was higher in both types of ASP (87.2–89.8 mg/100 g DW) than in wheat flour. The dietary fiber profile of the untreated ASP was comparable to that reported by Marques et al. [2013] who determined the SDF and IDF contents of 4.8 and 75.7%, respectively. Besides, the higher contents of ash in the powder of acerola fruit residue, consisting of seeds and peels (2.07%) and phytates in seed flour (230 mg/100 g DW) respectively, were previously reported [Marques et al., 2013; Sancho et al., 2015]. The differences could be due to the dissimilarities in acerola cultivars, the growing and processing conditions. Furthermore, the higher ash content of the treated ASP compared to that of the untreated one may be explained by using trisodium citrate buffer in the enzymatic process.
Table 1
Proximate composition, antioxidant contents, antioxidant capacity, and physical properties of untreated acerola seed powder (ASP), cellulase-treated ASP and wheat flour.
[i] Results are shown as mean ± standard deviation. Different letters in the same rows indicate significant differences (p<0.05). IDF, insoluble dietary fiber; SDF, soluble dietary fiber; TDF, total dietary fiber; DW, dry weight; DPPH•, 2,2-diphenyl-1-picrylhydrazyl radical; GAE, gallic acid equivalent; TE, Trolox equivalent; L*, lightness; a*, greenness (−) to redness (+); b*, blueness (−) to yellowness (+); ΔE, total color difference; ND, not detected.
The contents of ascorbic acid and total phenolics of the untreated ASP were 476 mg/100 g DW and 25.6 mg GAE/g DW, respectively (Table 1). Similar ascorbic acid content was found previously in acerola seed flour (457.32 mg/100 g DW) [Marques et al., 2013] and lower one in the powder from seeds and peels (170.73 mg/100 g) [Sancho et al., 2015]. The total phenolic content of ASP was higher than that in the range of 173.30–1155.2 mg GAE/100 g DW reported in different studies of the acerola fruit residue (seeds and peels) powder [Carvalho Gualberto et al., 2021; Sancho et al., 2015; Silva et al., 2021]. For the cellulasetreated ASP, the ascorbic acid content, total phenolic contents and the antioxidant capacity determined by DPPH and FRAP assays were reduced by 48, 7, 27 and 54%, respectively, as compared to those of the untreated counterpart (Table 1). A decrease in the total phenolic content and antioxidant capacity during the cellulase treatment and drying of dietary-fiber rich material was also reported in previous studies [Garau et al., 2007; Nguyen et al., 2022]. According to Garau et al. [2007], soluble dietary fiber and antioxidants may be modified or degraded during the extended air-drying period and/or high temperature.
It was noticed that the WHC and OHC of the untreated and cellulase-treated ASP were much higher than those of wheat flour (Table 1). A possible reason is related to the enormous amount of TDF in both ASP samples. The cellulolytic treatment caused a decrease in both WHC and OHC of ASP by 26 and 9%, respectively due to the lower IDF content. In comparison to SDF, IDF has a porous matrix structure formed by polysaccharide chains that can hold large amounts of water through hydrogen bonds [Zhu et al., 2010].
The results in Table 1 also reveal that redness (a* values) and yellowness (b* values) of both untreated and cellulase-treated ASP were higher, and that both powders were darker than wheat flour. The possible reason is that acerola seeds may contain color compounds.
Effects of partial replacement of wheat flour with acerola seed powder on the cookie quality
The chemical composition, antioxidant contents and antioxidant capacity of cookies produced from blends of wheat flour with untreated and cellulase-treated ASP are shown in Table 2. The use of untreated or cellulase-treated ASP in the recipe resulted in a slight enhancement of moisture, lipid, ash, and phytate contents in the cookies, but also in a considerable reduction of protein and starch contents. It can be explained by the significantly different composition between ASP and wheat flour (Table 1). The significantly higher moisture contents determined in the fortified cookies with 30% (w/w) replacement (AS30 and CAS30) compared to the control were due to the high WHC of dietary fiber in the supplement. At the same replacement levels, the differences between the cookies with cellulase-treated and untreated ASP were insignificant (p≥0.05) in terms of moisture, protein, lipid, and starch contents.
Table 2
Proximate composition, antioxidant contents and antioxidant capacity of cookies from wheat flour (control) and from blends of wheat flour with different proportion (10, 15, 20, 25 and 30%, w/w) of untreated acerola seed powder (AS10, AS15, AS20, AS25 and AS30, respectively) and cellulase-treated acerola seed powder (CAS10, CAS15, CAS20, and CAS30, respectively).
[i] Results are shown as mean ± standard deviation. In the same row, different lower-case letters (a–f) indicate significant differences between all cookie types, while upper-case letters (A and B) show significant differences between cookies produced with cellulase-treated and untreated acerola seed powder separately for each fortification level (p<0.05). IDF, insoluble dietary fiber; SDF, soluble dietary fiber; TDF, total dietary fiber; DW, dry weight; DPPH•, 2,2-diphenyl-1-picrylhydrazyl radical; GAE, gallic acid equivalent; TE, Trolox equivalent.
The use of ASP in the cookie recipe greatly improved its dietary fiber contents, specifically, at the 30% (w/w) replacement of wheat flour with ASP (Table 2). The TDF, IDF and SDF contents of the cookies produced from the flour blend with this level of replacement with the untreated ASP increased by 475, 822 and 29%, while those of the cookies with the treated counterpart were enhanced by 463, 711 and 143%, respectively, as compared to those of the control sample. The cookies produced from flour blends with 20, 25, and 30% (w/w) ASP were “high in fiber” food because their IDF contents were higher than 6 g/100 g [European Parliament & Council, 2006]. In the case of the developed cookies with the untreated ASP, an impressive increase in SDF content was not achieved compared to the control cookies (Table 2), because of the minor differences in SDF content between the untreated ASP (2.6 g/100 g DW) and wheat flour (1.6 g/100 g DW) (Table 1). However, the use of the cellulase-treated ASP led to 37–130% higher SDF content of these cookies compared to that of the control ones (Table 2). Interestingly, the enzymatic treatment successfully improved the ratio of IDF to SDF which was comparatively close to the recommendation for a well-balanced proportion to enhance the health benefit of both fiber fractions [Jha et al., 2017].
Ascorbic acid content, total phenolic content, and antioxidant capacity of the cookies increased significantly as the proportion of both untreated and cellulase-treated ASP was increased in the flour blends used to cookie production (Table 2). However, the cookies fortified with the cellulase-treated ASP had significantly (p<0.05) lower ascorbic acid content, total phenolic content, and antioxidant capacity than the cookies produced with an equal amount of the untreated ASP. A close correlation was observed in this study between the antioxidant capacity of cookies and their total phenolic content. The cookies produced from the flour blends with the 30% (w/w) of cellulase-treated and untreated ASP had a total phenolic content of 3.4 mg GAE/g DW and 3.1 mg GAE/g DW, respectively, whereas that determined in the control cookies was barely 0.7 mg GAE/g DW. The obtained results suggested that the use of ASP in cookies could elicit important health benefits.
The phytate content of the ASP-fortified cookies increased with the increasing proportion of ASP in the flour blend used in the production of cookies (Table 2). Similar values (p≥0.05) were found compared to the control cookies only for the AS10 and CAS 10 samples. The phytate contents did not differ significantly (p≥0.05) between the cookies with the untreated and cellulase-treated ASP (Table 2). It could be attributed to the significantly higher levels of phytates in the cellulase-treated and untreated ASP compared to that of wheat flour (Table 1). Phytates are an antinutrient that forms a chelate with minerals such as iron, calcium, copper, and zinc and prevents their bioavailability [Atuna et al., 2022].
The use of ASP significantly contributed to physical characteristics of the cookies. Their thickness and diameter decreased as the ratio of ASP in the flour blend increased, while the reverse result was observed for the spread ratio (Table 3). The diameters of the AS30 and CAS30 cookies decreased by 5.5 and 1.5%, respectively, and their thickness was reduced by 17 and 16%, respectively, but their spread ratios increased by 16 and 18%, respectively, compared to those of the control cookies. The decrease in cookie thickness was also reported in our previous study [Nguyen et al., 2021] for cookies with cellulase-treatment wheat bran. The reason could be the reduction of gluten content in the dough and the disruption of the gluten network, which is responsible for the vertical expansion of the cookie [Handa et al., 2012]. However, the contrary findings were found by Arun et al. [2015], who reported an increase in thickness of cookies with an increasing level of Nendran peel flour. It can be suggested that the addition of fiber reduces the amount of water available to dissolve soluble components in the cookie dough, increasing dough viscosity and reducing the spread of the product.
Table 3
Physical properties of cookies from wheat flour (control) and from blends of wheat flour with different proportion (10, 15, 20, 25 and 30%, w/w) of untreated acerola seed powder (AS10, AS15, AS20, AS25 and AS30, respectively) and cellulase-treated acerola seed powder (CAS10, CAS15, CAS20, and CAS30, respectively).
[i] Results are shown as mean ± standard deviation. In the same column, different lower-case letters (a–f) indicate significant differences between all cookie types, while upper-case letters (A and B) show significant differences between cookies produced with cellulase-treated and untreated acerola seed powder separately for each fortification level (p<0.05).
The cookies produced with the untreated ASP had a smaller (p<0.05) diameter and a higher (p<0.05) thickness compared to those of the cookies with the cellulase-treated ASP at the same replacement levels (Table 3). This could be related to the reduced IDF content since IDF has been proven to be unfavorable for the development of the gluten network [Foschia et al., 2015].
The use of ASP in the recipe led to an increase in the hardness and a decrease in the fracturability of the cookies (Table 3). However, the cookies produced from the flour blends with the cellulase-treated ASP (all proportions), interestingly, had lower (p<0.05) hardness and higher (p<0.05) fracturability than the samples with the untreated one. The CAS30 cookies showed 31% lower hardness and 11% higher fracturability than the AS30 samples. Previous studies reported that with increasing brewer’s spent grain addition to cookie recipe, the hardness of the final product increased [Heredia-Sandoval et al., 2020], while flaxseed flour-fortified cookies had decreasing hardness [Kaur et al., 2019]. The variations in the results may be caused by the different water absorption capacity of by-products. The increases in hardness could be due to the contribution of the high protein and IDF content, while the attenuation of hardness may be correlated with the competition between sugar and flour protein for water, which leads to the reduction of gluten formation [Morales-Polanco et al., 2017]. Future studies on interactions between wheat protein and dietary fiber of ASP are therefore essential to clarify the insight mechanisms that determine the physical properties of the final product.
The cookie color parameters and cookies appearance are shown in Table 4 and Figure 2, respectively. The cookie color became darker (lower L* values), more green (lower a values) and less yellow (lower b* values) with increasing levels of the untreated and cellulase-treated ASP. At the same proportion of both types of ASP in the flour blend, there were no differences in ΔE between the cookies with the cellulase-treated and untreated ASP except for the cookies incorporated with 25 and 30% (w/w); cookies with the cellulase-treated ASP had lower ΔE values than their counterparts with the untreated ASP. In general, the incorporation of higher-fiber ingredients usually causes a darker color of bakery products [Bolek, 2020]. Moreover, the natural pigment of ASP could contribute to the darkening of fortified cookies [Marques et al., 2015]. The Maillard reaction occurring between reducing sugars and amino acids, and caramelization of sugars might also be responsible for cookie color darkening [Žilić et al., 2021].
Figure 2
Appearance of cookies from wheat flour (control) and cookies from blends of wheat flour with different proportions (10, 15, 20, 25 and 30%, w/w) of untreated acerola seed powder (AS10, AS15, AS20, AS25 and AS30, respectively) and cellulase-treated acerola seed powder (CAS10, CAS15, CAS20, and CAS30, respectively).
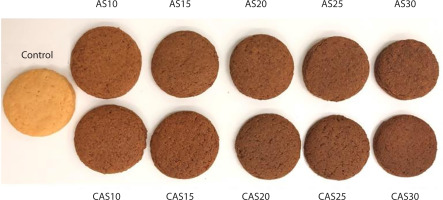
Table 4
The color parameters and overall acceptability of cookies from wheat flour (control) and from blends of wheat flour with different proportion (10, 15, 20, 25 and 30%, w/w) of untreated acerola seed powder (AS10, AS15, AS20, AS25 and AS30, respectively) and cellulase-treated acerola seed powder (CAS10, CAS15, CAS20, and CAS30, respectively).
[i] Results are shown as mean ± standard deviation. In the same column, different lower-case letters (a–f) indicate significant differences between all cookie types, while upper-case letters (A and B) show significant differences between cookies produced with cellulase-treated and untreated acerola seed powder separately for each fortification level (p<0.05); L*, lightness; a*, greenness (-) to redness (+); b*, blueness (-) to yellowness (+); ΔE, total color difference.”
The overall acceptability of the cookies fortified with different levels of both ASP types was significantly (p<0.05) higher than of the control cookies excluding the AS20, AS30 and CAS30 samples (Table 3). The previous study revealed that cereal bars with the addition of acerola seed flour were more preferred than the bars without the additive, because of the higher scores received for texture, flavor, overall appearance, and purchase intention [Marques et al., 2015]. However, the overall acceptability declined at high addition ratios (Table 4). The overall acceptability of the cookies was reduced as the fortified levels were 25% (w/w) or higher for the AS samples and 30% (w/w) for the CAS samples. The reduction in the sensory score of the cookies was due to the increased hardness, as the soft and crumbly texture is the most desirable characteristics of cookies [Lara et al., 2011].
CONCLUSIONS
The cellulase treatment was demonstrated to lower the IDF to SDF ratio of ASP owing to the conversion of IDF to SDF. The replacement of wheat flour with the cellulase-treated ASP in the recipe improved the IDF to SDF ratio of the cookies, making it close to the recommended value that efficiently provides health benefits. The cookies produced from the blend of wheat flour with the cellulase-treated ASP had a higher dietary fiber content and antioxidant capacity compared to the control cookies produced from wheat flour only. Moreover, the use of the cellulase-treated ASP at appropriate levels had a negligible effect on the geometric features of the fortified cookies, preserving their round shape and diameter. As compared with the cookies incorporated with the untreated ASP, the cookies prepared from the flour blend with the cellulase-treated ASP had lower hardness, higher fracturability, and generally higher overall acceptance. In the future, health effects of ASP-incorporated products, such as reduction in dietary postprandial glycemic response, should be investigated to confirm the potential of this by-product.