INTRODUCTION
Meat and meat products are nutrient-rich products usually exhibiting high water activity, making them susceptible to deterioration and spoilage. Their improper processing can lead to economic losses, food waste and loss of consumer confidence. The meat industry strives to meet growing consumer demand for high-quality raw meat and meat products with extended shelf-life. In this context, raw meat refers to meat that is stored fresh, uncooked, including cut or minced meat, with or without preservatives and not heat-treated.
High pressure processing (HPP) is a non-thermal preservation method most widely used in the food industry. Meat products account for nearly 25% of the market of foods produced using this technology [Bolumar et al., 2021]. The effect of pressure on the quality of meat and meat products has been described in numerous review papers [Bolumar et al., 2021; Cheftel & Culioli, 1997; Chuang & Sheen, 2022; Hygreeva & Pandey, 2016; Rajendran et al., 2022]. Raw meat cannot be pressurized under conditions that significantly reduce the microbial load (400–600 MPa), as this causes changes to meat colour [Bak et al., 2019; Jung et al., 2003]. An improvement in meat colour can be achieved at moderate pressures (130 MPa); however, processing in these conditions is not sufficient to reduce the number of meat microbiota [Jung et al., 2003].
Moderate pressures, from 20 to 200 MPa, are used in hyperbaric storage (HS). This innovative storage technique extends the shelf-life of raw meat without adverse changes in colour and may offer a promising alternative for the meat industry [Basso et al., 2022; Santos et al., 2020]. Santos et al. [2020] showed that the storage of beef and pork meat for 60 days at pressures of 50–100 MPa at room temperature had little effect on their colour. At pressures higher than 50 MPa, the growth of meat microbiota was also inhibited or inactivated. Unfortunately, storage at higher pressures (≤75 MPa) promoted the formation of secondary products of meat lipid oxidation which additionally occurred faster than during refrigerated storage at atmospheric pressure [Santos et al., 2021]. It is likely that lowering the HS temperature can lead to slower oxidation processes. Such conditions of pressure and subzero temperature, in which the water remains unfrozen, seem particularly interesting. To the best of our knowledge, there are very limited data on changes in food stored at moderate pressures and subzero temperatures. From an economic point of view, comparing HS-ST to conventional frozen storage, the former method allows for significant energy savings as storage can be carried out at a higher temperature than is possible at atmospheric pressure. Moreover, since water does not crystallise under HS-ST, the cold demand (energy required for cooling) will be much lower than in conventional freezing, where the cold demand to freeze a food product includes the amount of heat that must be removed from the product as a result of lowering the initial temperature to the cryoscopic temperature (cooling), the phase transformation of water into ice (freezing proper) and the lowering of the temperature of the frozen product from the cryoscopic temperature to the final freezing temperature. With lower energy requirements, the HS-ST represents a more environmentally friendly alternative to conventional frozen storage.
The aim of our study was to determine the selected quality characteristics and shelf-life of raw pork sausages during hyperbaric storage under moderate pressures (60 and 111 MPa) in subzero temperatures (without freezing water) for a long time (up to 35 days).
MATERIALS AND METHODS
Sample preparation
The raw sausages used in experiments were purchased from a local manufacturer and consisted of pork meat (82 g/100 g), water, flavours, salt, glucose, spices, spice extracts, acidity regulator: sodium citrate, yeast extract and garlic extract. Final products were portioned and packed in protective atmosphere by the producer and transported to company store. According to producer’s declaration, their shelf-life from manufacture was 7 days under refrigeration. The raw material was transported from market to the laboratory with a cold chain continuity and then unpacked from collective packaging and divided for single portions (about 130 g) which were vacuum-packed in commercially available plastic bags characterized by high barrier properties to oxygen and to water vapor (polyamide/linear low-density polyethylene, Food Saver, Gdynia, Poland, cat. no. 72459) using vacuum packaging machine (Hendi, De Klomp, The Netherlands).
Hyperbaric storage
Hyperbaric storage was performed using equipment designed at the Department of Food Chemistry, Technology and Biotechnology, Gdańsk University of Technology (Poland) and built by DS-Technology Ltd. (Słupsk, Poland). Pressure was generated as described in our previous publication [Malinowska-Pańczyk et al., 2014]. The raw sausages were subsequently stored at 60 MPa/−5°C and 111 MPa/−10°C. The conditions of hyperbaric storage at subzero temperature (HS-ST) were selected based on previous research results in which no changes were found in the properties of raw pork meat after HPP at subzero temperature [Malinowska-Pańczyk et al., 2014]. Samples were placed in 620 mL pressure vessels that were filled with distilled water, next secured with a spring that kept the sample in the upper unfrozen zone and then sealed tightly. The pressure vessel was placed from the side of the closure in a cryogenic bath and gradually immersed to cool the system and generate pressure. The total time for this step was 90 min. After this time, the pressure vessels were transferred to a storage section and kept therein for up to 35 days. The magnitude of the pressure generated in such a system depends on the temperature [Bridgman, 1912; Rubinsky et al., 2005]. Simultaneously samples were kept at 4, −5°C and −10°C at 0.1 MPa and were used as controls (15 samples for each condition). Before analyses, the samples were thawed at 0–2°C.
Microbial analysis
Microbial enumerations were performed after 1, 3, 7, 10 and 14 days of storage at 4°C for the control samples. In the case of raw sausages treated with HS-ST and sausages stored at −5°C and −10°C at 0.1 MPa, analysis was carried out after 3, 7, 14, 21, and 35 days of storage. Minced samples (10 g) were transferred to a stomacher bag and homogenised in a stomacher (Masticator Basic Panoramic, IUL Instruments, Barcelona, Spain), with 90 mL of saline peptone water for 1 min. Decimal dilutions were also prepared in saline peptone water and then plated (in duplicate) onto an appropriate agar medium and incubated (Table 1). Cultures from dilutions that contained between 10 and 300 colony forming units (CFU) were selected for colony counting. The number of coagulase-positive staphylococci was determined in accordance with the standard established by the International Organization for Standardization (ISO) [PN-EN ISO 6888-1:2022]. The media were purchased from Merck KGaA (Darmstadt, Germany). Microbial counts were expressed as log CFU/g of stored raw sausages.
Table 1
Media and incubation conditions used during microbial analysis.
Determination of thiobarbituric acid reactive substance content
Thiobarbituric acid reactive substance (TBARS) content was determined as described by Salih et al. [1987] with a small modification where butylhydroxytoluene (BHT) was used instead of butylhydroxyanisole. To 10 g of minced sausages after storage, 34.25 mL of 4% (v/v) perchloric acid at 4°C and 0.75 mL of 0.01% BHT solution were added. The sample was homogenised at 10,000 rpm for 2 min using Silent Crusher M (Heidolph, Schwabach, Germany). The homogenate was filtered through Whatman paper No. 1. The filtrate was made up to 50 mL by washing the precipitate on the filter with 4% (v/v) perchloric acid. Five millilitres of a 0.02 M aqueous solution of 2-thiobarbituric acid (TBA) were added to 5 mL of the filtrate, which was then boiled for 1 h. Afterwards, the samples were cooled in a cold, tap water. Absorbance at 532 nm was then measured against a blank containing 5 mL of 4% (v/v) perchloric acid instead of filtrate. The TBARS content was calculated from the standard curve and expressed as mg of malondialdehyde (MDA) equivalent in 1 kg of sausage. Determinations of TBARS content in the control samples stored at 4°C were carried out on days 1, 10, 14 and 17, whereas in the samples stored at subzero temperature under atmospheric and moderate pressure on days 5, 14, 21 and 35.
Determination of protein solubility
The solubility of protein in sausages before storage and stored for 5, 7, 14, 21 and 35 days at 60 MPa/−5°C and 111 MPa/−10°C or at −5°C and −10°C at 0.1 MPa was assayed. The minced sample was homogenised in 0.9 M saline at pH 7.5 for 5 s at 7,000 rpm followed by 30 s at 12,000 rpm using Silent Crusher M (Heidolph) [Malinowska-Pańczyk et al., 2014]. The homogenate was centrifuged at 4,500×g for 30 min at 4°C. The biuret method was used to determine the concentration of proteins (mg) in 1 mL of the supernatant [Gornall et al., 1949]. Relative protein solubility (%) was calculated from the ratio of soluble protein determined in the control initial samples to the soluble protein content of the stored samples.
Drip loss determination
Drip loss was calculated as the percentage ratio of the weight of the sample after 5, 7, 14, 21 and 35 days of storage at atmospheric (0.1 MPa) or moderate pressure to the initial weight, according to 1:
where: DL, drip loss (%); m1, the weight of the sample before storage (g); and m2, the weight of the sample after storage (g).Statistical analysis
The storage experiments were conducted on three different batches of raw sausage from a single manufacturer. Determinations of microbial and physicochemical parameters were performed in triplicate. The results in the figures are presented as mean value and standard deviation (SD). To test the statistical significance of the observed differences in selected qualitative traits (TBARS content, degree of protein denaturation, drip loss) and microbiota count between samples stored at different times and different temperatures under atmospheric and moderate pressure conditions, a two-way analysis of variance (ANOVA) was conducted. Differences were considered statistically significant at p<0.05. Differences between groups were determined with a Tukey test. The statistical analysis was conducted using Prism software, version 10.2.0 (GraphPad Software, Inc., San Diego, CA, USA).
RESULTS AND DISCUSSION
The effect of hyperbaric storage at subzero temperature on the microbial quality of sausages
Sausages sold in the raw form are the type of the meat products for which the European Communities (EC) Commission Regulation on Microbiological Criteria for Foodstuffs 2073/2005 established that the upper limit for Escherichia coli and S. aureus should not exceed 3.7 log CFU/g (5×103 CFU/g) [Commission Regulation (EC), 2005]. E. coli were not detected in the control sausages (data not shown), which indicates good hygienic conditions during production and distribution. The number of coagulase-positive staphylococci in raw sausages before storage was 3.0 log CFU/g (Figure 1D). It also did not exceed the upper limit established by the above-mentioned EC Commission Regulation. Because the total microbial load in meat determines its shelf-life and spoilage [Fougy et al., 2016; Vasilopoulos et al., 2015], the total bacteria count (TBC), psychrophilic bacteria count (PBC) and coliform count were additionally determined in the study. The initial TBC in the unpressurised samples was 4.3 log CFU/g (Figure 1A). This level of microbial contamination indicated a good product quality. Psychrophilic bacteria were also present in the samples, at 3.9 log CFU/g (Figure 1B).
Figure 1
Total bacteria count (A), psychrophilic bacteria count (B), coliform count (C), and coagulase-positive staphylococci count (D) of raw pork sausages stored at different temperatures under atmospheric and moderate pressure conditions. The results are shown as mean and standard deviation. The different lowercase letters (a–f) indicate the significant differences among different storage times for the same storage conditions, and the different capital letters (A–E) indicate the significant differences among different storage conditions at the same time (p<0.05). The dashed line indicates the detection limit.
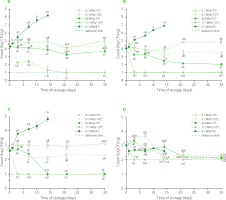
When the samples were stored under refrigeration, it was found that TBC and PBC increased in the control samples (Figure 1A and 1B). TBC exceeded the upper acceptable limit (<6.7 log CFU/g) on the 7th day of storage. After 14 days, the TBC and PBC in the control samples were at ca. 8 and 7 log CFU/g, respectively. TBC in sausages stored for 35 days under 60 MPa/−5°C decreased only slightly (0.6 log cycle). However, at 111 MPa/−10°C, TBC lowered to an undetectable level after 21 days. In the samples stored at 0.1 MPa/−5°C and 0.1 MPa/−10°C at the same time, the TBC did not change significantly (p≥0.05). Santos et al. [2020] showed that storage of pork and beef meat at 50 MPa at room temperature not only did not inhibit the growth of total aerobic mesophiles but even increased the number of microbial populations. Inactivation of the meat microbiota was achieved only during storage at higher pressures of 75 and 100 MPa [Santos et al., 2020]. PBC decreased to 2 log cycles in the samples stored at 60 MPa/−5°C for 35 days and was not detected after 3 days of storage at 111 MPa/−10°C (Figure 1B). A reduction of psychrophilic microbiota by about 1.25 log cycle in fresh salmon stored at 60 MPa/10°C for 50 days was also observed by Fidalgo et al. [2019].
During storage of control sausages for 14 days in chilled conditions, the number of coliforms increased by 2.3 log cycles (Figure 1C). HS-ST of sausages caused a successive decrease in the count of coliforms, which were not detected after the 3rd and 14th day of storage at 111 MPa/−10°C and 60 MPa/−5°C, respectively. In the case of the samples stored at 0.1 MPa/−5°C, the number of coliforms increased by 0.5 log cycle, while in those stored at 0.1 MPa/−10°C it decreased by 0.3 log cycle. However, these changes were not statistically significant (p≥0.05).
The population of coagulase-positive staphylococci did not change (p≥0.05) throughout the storage period of the control samples at 4°C (Figure 1D). In the case of the sausages stored under pressure at subzero temperature, they were not detected after 3 days of storage at 111 MPa/−10°C, whereas in these stored at 60 MPa/−5°C, their number decreased successively. A reduction in the number of coagulase-positive staphylococci was also observed in the samples stored at analogous temperatures at atmospheric pressure.
The effect of hyperbaric storage at subzero temperature on the content of thiobarbituric acid reactive substance in sausages
The main factor in non-bacterial spoilage of meat and meat products that leads to the loss of nutritional value is lipid oxidation. Secondary products formed during this reaction readily react with proteins, causing changes in the colour, aroma, and taste of raw meat [Fuentes et al., 2010]. Lipid oxidation in meat can be initiated endogenously via metal ions, especially haeme iron, and by exogenous reactive oxygen species [Amaral et al., 2018]. HPP can promote lipid oxidation reactions [Cheah & Ledward, 1996]. Pressure causes conformational changes in haemoproteins and leads to the release of iron from haeme, which induces lipid oxidation [Beltran et al., 2003; Rajendran et al., 2022].
The initial level of secondary lipid oxidation products in the raw sausages was 0.15 mg MDA/kg (Figure 2), which indicates the good quality of the raw materials and the maintenance of appropriate conditions during sausage stuffing production. A successive increase in TBARS value was found in the control samples during refrigerated storage. After 17 days of storage, the TBARS content in these samples was 0.63 mg MDA/kg.
Figure 2
Content of thiobarbituric acid reactive substances (TBARS) in raw pork sausages stored at different temperatures under atmospheric and moderate pressure conditions. The results are shown as mean and standard deviation. The different lowercase letters (a–d) indicate the significant differences among different storage times for the same storage conditions, and the different capital letters (A–C) indicate the significant differences between different storage conditions at the same time (p<0.05).
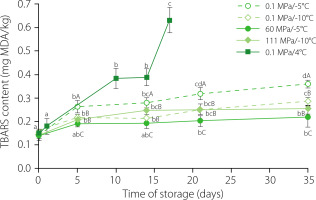
The content of secondary metabolites of lipid oxidation in the samples stored at subzero temperatures increased slightly (Figure 2). The TBARS content of the samples stored at 0.1 MPa/−5°C was higher on each day of storage compared to the samples stored at the same temperature under 60 MPa/−5°C (p<0.05). For sausages stored at −10°C, there were no differences between TBARS content in sausages stored at 0.1 and 111 MPa (p≥0.05). After 35 days of storage at 0.1 MPa/−5°C and 0.1 MPa/−10°C, it was 0.36 and 0.28 mg MDA/kg sausages, respectively. In the case of the samples stored at 60 MPa/−5°C and 111 MPa/−10°C, the TBARS values determined on the last day of storage were 0.23 and 0.26 mg MDA/kg sausages, respectively. The difference in the TBARS content between the samples at the beginning and end of HS-ST was significant (p<0.05). The TBARS content of sausages stored for 35 days at subzero temperatures both at moderate and atmospheric pressure was considerably lower than of the control sausages stored under refrigeration for 17 days. A rancid odour and taste of pork is detected by consumers even when the TBARS value is 0.5–1.0 mg MDA/kg [Wood et al., 2008]. Only in the control sausages stored at 4°C did TBARS levels exceed the threshold value. The data showed that HS-ST effectively retarded lipid oxidation in raw meat products. Such a phenomenon was not observed in pork (in pieces) and beef (minced) stored for 60 days at 60 and 75 MPa at 10 and 25°C, respectively [Santos et al., 2021]. The authors showed that under these conditions, the formation of secondary lipid oxidation products occurred more rapidly than in meat stored under refrigeration at atmospheric pressure. It seems that one of the main factors affecting the rate of meat lipid oxidation during hyperbaric storage is the temperature of the process. During storage at atmospheric pressure, oxidation occurs more slowly the lower the process temperature is [Shimizu & Iwamoto, 2022]. Probably, the oxidation rate is less dependent on the value of pressure, but this relationship needs to be confirmed.
The effect of hyperbaric storage at subzero temperature on denaturation of proteins and drip loss of sausages
Figure 3 shows changes in protein solubility and drip loss of sausages after HS-ST. It was noticed that protein solubility, which is an indicator of the degree of protein denaturation, depended on pressure value and time of exposure. A successive decrease in protein solubility was determined in the samples stored at 60 MPa/−5°C, to 21.5% on the 35th day of storage compared to the samples before storage. Still lower protein solubility was observed in the samples stored at 111 MPa/−10°C. Already after 5 days of storage, the solubility decreased by 57.5% compared to that of raw sausages before storage and did not change (p≥0.05) during further storage. For the samples stored at −5°C and −10°C at atmospheric pressure, there were no significant changes (p≥0.05) in solubility over the storage period. Additionally, greater level of denaturation was correlated with a higher drip loss (Figure 3B). According to Fernández et al. [2007], water is less retained in pressure-treated meat due to the denaturation of myofibrillar proteins induced under these conditions. Drip loss increased even in the first five days of hyperbaric storage, and then remained at a constant level. The greatest changes were observed in the samples stored at 111 MPa/−10°C.
Figure 3
The relative solubility of proteins (A) and drip loss (B) in raw pork sausages stored at different temperatures under atmospheric and moderate pressure conditions. The results are shown as mean and standard deviation. The different lowercase letters (a–b) indicate the significant differences among different storage times for the same storage conditions, and the different capital letters (A–B) indicate the significant differences between different storage conditions at the same time (p<0.05).
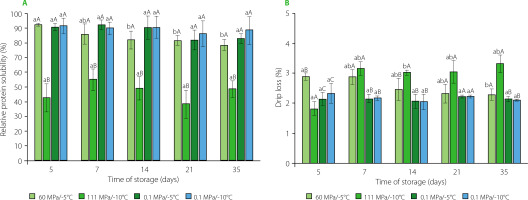
Changes were also observed after 35 days of storage in the appearance and colour of the samples (Figure 4), particularly in the samples stored at 111 MPa/−10°C with a shift in colour from pink to greyish-pink or grey. According to Ludikhuyze et al. [2001], a reduction in the colour intensity of meat subjected to 200–350 MPa treatment occurs due to the denaturation of globin, or the displacement or release of haeme from the myoglobin molecule.
CONCLUSIONS
The study showed HS-ST to be a useful technique to extend the shelf-life of raw sausages. Under these conditions, the growth of the microbiota was inhibited, or the population numbers decreased with the extension of the storage period. Sausage storage at 60 MPa/−10°C significantly reduced the formation of secondary lipid oxidation products. Such a phenomenon was not found during storage of the samples at 111 MPa/−10°C and at −5°C and −10°C at atmospheric pressure. A negative phenomenon observed during storage at 111 MPa/−10°C was a change in the colour of sausages that was noticeable to the naked eye. In addition, during HS-ST (111 MPa/−10°C), there was an intense drip loss, which means not only financial losses related to such meat, but also the loss of valuable ingredients such as vitamins, mineral salts or water. Our research shows that HS-ST can be an effective method for extending the shelf-life of raw meat without drastic changes in quality, but the applied pressure must be less than 100 MPa.
Future research will provide knowledge of the phenomena that occur during storage at moderate pressure and subzero temperature, which will allow the full potential of HS not only to extend the shelf-life of meat, but also to adapt certain properties of meat raw materials to develop new meat products. The latter aspect is so far completely unexplored but represents a very promising research topic. The ability to track the transformation of components during storage of muscle tissue at temperatures below 0°C without freezing the water will bring new elements to the existing knowledge of the phenomena occurring in meat at low temperature, including under moderate pressure, and additionally perhaps enable the verification of some hypotheses regarding the denaturation of muscle proteins induced by freezing.