INTRODUCTION
Radish sprouts have gained popularity worldwide due to their nutritional value and a high content of bioactive compounds including flavonoids, phenolic acids, glucosinolates, isothiocyanates, and ascorbic acid [Gamba et al., 2021]. Their ingestion may elicit a hypoglycemic effect by lowering the plasma levels of fructosamine and glucose followed by a decrease in the plasma level of insulin and improved insulin sensitivity. Radish sprouts are known to exhibit high antioxidant activity and to lower the risk of development of certain cancers and heart diseases [Abellán et al., 2019]. Moreover, health-promoting phytochemicals are more concentrated in sprouts than in adult plant edible organs [Gamba et al., 2021].
Although consumption of sprouts has been associated with health benefits, they have also been claimed to be one of the most common sources of bacterial foodborne illnesses. There have been documented multiple outbreaks of foodborne diseases induced by sprout consumption, with the causative agents being primarily Shiga toxin-producing Escherichia coli and various serotypes of Salmonella [Miyahira & Antunes, 2021; NACMCF, 1999]. According to the Centers for Disease Control and Prevention (CDC) and U.S. Food and Drug Administration (FDA) data [CDC, 2023; FDA, 2023], there have been ten sprouts-related outbreaks noted over the last 10 years, in which a total of 367 people were infected and 77 people required hospitalization. In a Listeria monocytogenes outbreak linked to mung bean sprouts in 2014, two of the five hospitalized people died, while no deaths were reported in other outbreaks.
The other pathogens most frequently isolated from sprouted seeds were Pseudomonas aeruginosa, Staphylococcus aureus, and Bacillus cereus [Kang et al., 2011; NACMCF, 1999; Viswanathan & Kaur, 2001]. Contaminated seeds have been recognized to be the main source of pathogenic bacteria introduced into sprouts [Miyahira & Antunes, 2021; NACMCF, 1999; Sikin et al., 2013]. Seeds usually harbour native aerobic microbiota (102–107 CFU/g) and fecal coliforms (102–103 CFU/g) [Michalczyk & Kowalińska, 2009; Sikin et al., 2013]. However, during sprouting the number of bacteria can increase significantly, reaching from >106 to even 1012 CFU/g [Lang et al., 2016; Miyahira & Antunes, 2021; NACMCF, 1999; Sikin et al., 2013].
In order to reduce the risk posed to the consumer by the consumption of contaminated sprouts, various methods of seeds disinfection have been applied. It is known that disinfection of seeds prior to sprouting is more effective than that of sprouts [Sikin et al., 2013]. According to FDA recommendations [FDA, 2023], soaking seeds in 20,000 mg/L of a chlorine solution before sprouting should be applied as a preventive method. However, this method has been proved poorly effective in eliminating Salmonella spp. and E. coli O157:H7 [Montville & Schaffner, 2004]. Moreover, the use of chlorine as a disinfectant can result in the formation of carcinogenic compounds and the emergence of new, more tolerant pathogens [Allende et al., 2008]. The use of 20,000 mg/L calcium hypochlorite may also pose a risk to the environment and worker safety. Therefore, new, safe and effective methods are being sought to disinfect seeds prior to sprouting and thus enhance consumer safety.
Sea buckthorn (Hippophae rhamnoides L.) has been used in traditional medicine by Tibetans and Mongolians for over 2,000 years [Chen et al., 2023]. Currently, the most important area of its application, apart from the production of pharmaceuticals, is food and feed production. The plant, especially its berries, is used as a raw material to produce functional food or food supplements. It can also be successfully provided to consumers who promote a healthy lifestyle or those who are interested in preventing or alleviating diet-related diseases [Chen et al., 2023; Jaśniewska & Diowksz, 2021]. Sea buckthorn berries are valued for their high content of various essential nutrients, such as essential amino acids, polyunsaturated fatty acids (especially omega-7 palmitoleic acid), vitamins (mainly vitamin C and E), minerals and a variety of bioactive compounds with flavonoids and phytosterols being the main medicinal active ingredients [Chen et al., 2023]. The health benefits for the consumer are related to antimicrobial activity of the berries, their anti-inflammatory effects, support of tissue regeneration, protection against cancer, strengthening the immune system, as well as anti-myocardial, anti-hyperlipidemic, fatty liver-preventing, and wound-healing effects [Jaśniewska & Diowksz, 2021]. Sea buckthorn fruit, as a rich source of many active substances with health-promoting properties, seems to be a valuable material for use in a broad range of products, as available in some countries fruit vinegar, sea buckthorn yogurt, sea buckthorn fermented soy milk, sea buckthorn nutritional milk powder, sea buckthorn probiotic yogurt or probiotic drink, and others [Chen et al., 2023].
This study was undertaken to determine the efficacy of the extract from dried sea buckthorn fruit in the disinfection of contaminated radish seeds in comparison to the treatment with 20,000 mg/L active chlorine. Previous studies have shown seed germination to be the critical moment from the microbiological viewpoint, and sprouting conditions to promote bacteria development [NACMCF, 1999; Sikin et al., 2013]. Hence, the efficacy of 15-min treatment as well as 3-h soaking of the seeds in the extract was evaluated in this study.
MATERIALS AND METHODS
Preparation of sea buckthorn fruit extracts
The dried sea buckthorn fruit (Dary Podlasia Adam Nowicki, Bielsko Podlaskie, Poland) were ground to powder, and 10 g of the powder were suspended in 90 mL of water and in 90 mL of 5% (v/v) ethanol to obtain water and water-ethanol extract, respectively. Extraction was carried out for 40 min in an ultrasonication bath (Sonorex, Bandelin Electronic, Berlin, Germany) at 40°C [Rajkowska et al., 2023]. After cooling, the samples were filtered through a Whatman filter paper (grade 1:11 μm) and centrifuged at 2,800×g for 10 min. In order to obtain sterile extracts, supernatants were filtered using Minisart syringe filters with a pore size of 0.45 μm (Sartorius Stedim Biotech, Goettingen, Germany). The extracts were stored at 4°C in the dark up to 1 month.
Assessment of antibacterial activity
Antibacterial activity of water and water-ethanol extracts of sea buckthorn fruit, and a water solution of calcium hypochlorite (Warchem Sp. z o.o., Zakręt, Poland) at a concentration of 20,000 mg/L was determined with the agar diffusion plate method using the following bacterial strains: Escherichia coli ATCC 8739, Salmonella enterica subsp. enterica serovar Enteritidis ATCC 13076, Listeria monocytogenes ATCC 19111, and Staphylococcus aureus ATCC 6538 (purchased from Merck Life Science Sp z o.o., Poznań, Poland). One hundred microliters of a bacterial suspensions (107–108 cells/mL) in a sterile saline solution (sodium chloride 8.5 g/L) were spread on the surface of Tryptic Soy Agar (TSA) (pancreatic digest of casein 15 g/L, papain digest of soya 5 g/L, sodium chloride 5 g/L, agar 15 g/L; pH 7.2; Merck KGaA, Darmstadt, Germany). Then, blank antimicrobial susceptibility discs (Ø 6 mm, Thermo Fisher Scientific, Waltham, MA, USA) were placed centrally on the plates, and 20 μL of the tested substances were applied. In addition, the antibacterial effect of 5% (v/v) ethanol was tested as a control for the water-ethanol extract. The plates were incubated at 37°C for 24 h. The results were expressed as bacterial growth inhibition zones around the discs (mm) as an arithmetic mean from three independent experiments.
Inoculation and disinfection of contaminated radish seeds
The bacterial suspensions were prepared in a sterile saline solution from TSA slants cultures of the tested microorganisms to achieve a final inoculum containing 108 to 109 cells/mL, according to McFarland standards. Radish seeds for sprouts and microgreens were obtained from a commercial supplier (Zielone Witaminy Kamil Stankiewicz, Lidzbark, Poland) and stored at room temperature until use. Radish seeds (100 g) were added to the inoculum of the tested bacteria monocultures (150 mL) and gently mixed for 2 min. The seeds were drained, spread over double-layer of sterile Miracloth (Calbiochem, San Diego, CA, USA) on the top of a plastic rack, and air-dried for 48 h under a laminar flow hood (Airstream, Esco Lifesciences, Friedberg, Germany).
Ten grams of seeds contaminated with bacteria were submerged with 25 mL of sterile distilled water (control), 20,000 mg/L calcium hypochlorite solution or sea buckthorn fruit extracts for 15 min at room temperature with gentle agitation on a platform shaker (100 rpm). The seeds were drained and those treated with calcium hypochlorite were rinsed in 100 mL of distilled water.
The efficacy of disinfection after seed soaking, which is the standard procedure for seeds before germination, was also assessed. Seeds previously treated with calcium hypochlorite were soaked in sterile distilled water, and those treated with the sea buckthorn fruit extract were soaked in the same extract, for 3 h at room temperature. Seeds soaked in sterile distilled water served as a control.
Enumeration of microorganisms
The number of bacteria was determined before and after treatment with calcium hypochlorite, sea buckthorn fruit extract, and water as control, as well as after soaking. Ten grams of seeds were weighed and transferred to 90 mL of a peptone (1.0 g/L) water solution (after treatment with water and sea buckthorn fruit extract) or to 90 mL of a peptone (1.0 g/L) water solution with the addition of 37.0 g/L sodium thiosulfate as a neutralizer [Jeong et al., 2010]. The samples were homogenized in a stomacher (Devimix, De Ville Biotechnology, Raszyn, Poland) for 2 min, and serial tenfold dilutions were prepared. Enumeration of bacteria was performed by the pour plate method using selective media, i.e., Tryptone-Bile-X-Glucuronate (TBX) agar (bile salts 1.5 g/L, X-β-d-glucuronide 0.075 g/L, peptone 20.0 g/L, agar 15.0 g/L; pH 7.2; Merck KGaA, Darmstadt, Germany) for E. coli, Xylose Lysine Deoxycholate (XLD) agar (ferric ammonium citrate 0.8 g/L, lactose 7.5 g/L, l-lysine hydrochloride 5.0 g/L, phenol red 0.08 g/L, sodium chloride 5.0 g/L, sodium deoxycholate 1.0 g/L, sodium thiosulfate 6.8 g/L, sucrose 7.5 g/L, xylose 3.75 g/L, yeast extract 3.0 g/L, agar 15.0 g/L; pH 7.4; Merck KGaA, Darmstadt, Germany) for S. enterica, Listeria mono Differential Agar Base according to Ottaviani & Agosti (casein enzymic hydrolysate 6.0 g/L, 5-bromo-4-chloro-3-indolyl-β-d-glucopyranoside 0.05 g/L, disodium hydrogen phosphate anhydrous 2.5 g/L, glucose 2.0 g/L, lithium chloride 10.0 g/L, magnesium glycerophosphate 1.0 g/L, magnesium sulfate 0.5 g/L, meat peptone 18.0 g/L, sodium chloride 5.0 g/L, sodium pyruvate 2.0 g/L, yeast extract 10.0 g/L, L-α- -phosphatidylinositol 0.016 g/L, selective supplement 40 mL/L: nalidixic acid 0.02 g/L, ceftazidime 0.02 g/L, amphotericin B 0.01 g/L, and agar 15.0 g/L; pH 7.2; Merck KGaA, Darmstadt, Germany) for L. monocytogenes, and Baird-Parker agar (casein peptone 10.0 g/L, glycine 12.0 g/L, lithium chloride 5.0 g/L, meat extract 5.0 g/L, sodium pyruvate 10.0 g/L, yeast extract 1.0 g/L, egg yolk emulsion 50 mL/L, agar 15.0 g/L; pH 6.8; Merck KGaA, Darmstadt, Germany) for S. aureus. The plates were incubated at 37°C for 48 h. The number of colony forming units per 1 g of seeds (CFU/g) was counted.
On the basis of the number of bacteria after treatment in water as control (Ncontrol) and after treatment with the tested substances (Ntest), the decontamination effect was calculated as microbial reduction percentages, according to equation (1) [Mascarenhas et al., 2022]:
Radish seed germination
Uninoculated radish seeds treated with water, calcium hypochlorite, and water sea buckthorn extract for 15 min as described above, were placed on sterile moistened filter pad (Whatman, grade 1:11 μm) in sterile Petri dishes (140×20 mm, Biospace, Poznań, Poland). The filter paper was moistened with 10 mL of distilled water once a day or more frequently if necessary [Charkowski et al., 2001]. The percentage of seeds that germinated at room temperature (22–24°C) after 24, 48, 72 and 96 h was determined. The length of hypocotyls and roots of all sprouts was also measured after 96 h. A total of 100 radish seeds were evaluated in triplicate.
High-performance liquid chromatography analysis of sea buckthorn fruit extract
Chromatographic separation of phenolic compounds of the sea buckthorn fruit extract was carried out according to Czyżowska et al. [2020] on a Spherisorb ODS2 column (250×4.6 mm, 5 μm packing) (Waters, Milford, MA, USA). The mobile phase consisted of 5% formic acid (solvent A) and 95% acetonitrile (solvent B). The samples were eluted with the following gradient: 2 min, 97% (A); 13 min, 97–85% (A); 9 min, 85–82% (A); 31 min, 82–75% (A); 5 min, 75–70% (A). The flow rate was 0.8 mL/min, and the injection volume was 10 μL of the standard solution or extract. Analyses were performed using a Finnigan Surveyor high-performance liquid chromatography (HPLC) system equipped with an autosampler and a diode array detector (Thermo Scientific, Waltham, MA, USA). Phenolic compounds were quantified according to calibration curves established, at specific wavelengths, for gallic acid (280 nm), caffeic acid (320 nm), and quercetin-glucoside (360 nm). The content of compounds with λmax closest to 280 nm was expressed as gallic acid equivalent, the content of compounds with λmax closest to 320 nm was expressed as caffeic acid equivalent and flavonols as quercetin-glucoside equivalent. The results were expressed as mg/L of the extract.
Compounds were identified using available standards and based on the literature [Guo et al., 2017; Wang et al., 2022]. UV absorption spectra were scanned in the range of 210–500 nm. The peaks were identified by comparisons of the UV absorption spectra to those of the reference compounds. Most standards (gallic acid, protocatechuic acid, p-hydroxybenzoic acid, vanillic acid, ferulic acid) were purchased in Sigma-Aldrich/Merck (Poznań, Poland). Quercetin 3-rutinoside, isorhamnetin 3-rutinoside, isorhamnetin 3-glucoside, and kaempferol 3-rutinoside were purchased from Extrasynthese (Genay, France).
Statistical analysis
The results were expressed as the arithmetic mean and standard deviation from three independent experiments. The significance of differences between means was determined using analysis of variance (one-way ANOVA; OriginPro 8.1, OriginLab Corporation, Northampton, MA, USA) and Tukey’s Honest Significant Difference (HSD) test, with p<0.05.
RESULTS AND DISCUSSION
Antibacterial activity of sea buckthorn fruit extracts
Initially, the antibacterial potential of water and water-ethanol extracts from dried sea buckthorn fruit was determined using the disc diffusion method, compared to the recommended calcium hypochlorite solution at a concentration of 20,000 mg/L. The assay was performed against selected strains of bacteria infecting sprouts and posing a potential health hazard to the consumer [Fleckenstein et al., 2021; Miyahira & Antunes, 2021; NACMCF, 1999; Viswanathan & Kaur, 2001], i.e., E. coli, S. enterica, L. monocytogenes, and S. aureus. All tested substances showed antibacterial activity against these strains (Figure 1). Calcium hypochlorite elicited a significantly higher inhibitory effect on the growth of E. coli, S. enterica, and L. monocytogenes. However, sea buckthorn water extract was comparably effective against S. aureus and, concurrently, produced the largest growth inhibition zone (15.3 mm) compared to other tested bacteria (7.8 mm for E. coli, 8.8 mm for S. enetrica, and 8.9 mm for L. monocytogenes). Due to the significantly higher antibacterial activity of the water extract (growth inhibition zones of 7.8–15.3 mm) than the water- -ethanol extract (6.8–11.0 mm), the water extract of sea buckthorn fruit was used in further studies on radish sprouts contaminated with bacteria.
Figure 1
Photographic images of disc diffusion test results with values of zones of bacterial growth inhibition (mm) after treatment with calcium hypochlorite at a concentration of 20,000 mg/L, water extract and water-ethanol extract from sea buckthorn fruit. Means followed by different letters differ significantly (p<0.05).
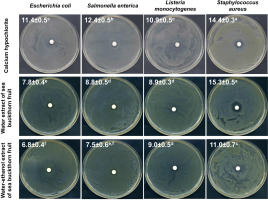
The results obtained in this study are consistent with reports on the high antimicrobial activity of water and methanolic sea buckthorn extracts against S. aureus, Bacillus subtilis, Streptococcus pneumoniae, Pseudomonas aeruginosa, E. coli, and Klebsiella pneumoniae [Chaman et al., 2011]. Ivanišová et al. [2020] showed that also other products of Hippophae rhamnoides, i.e., oil, juice, dried berries and tea, had strong antimicrobial activity against E. coli, S. enterica, Bacillus thuringiensis, L. monocytogenes, S. aureus, and especially Yersinia enterocolitica. Sea buckthorn leaves also seem to be a valuable source of compounds with antibacterial activity, and both aqueous and hydroalcoholic leaf extracts inhibited the growth of Bacillus cereus, P. aeruginosa, S. aureus, and Enterococcus faecalis [Upadhyay et al., 2010]. The antimicrobial properties of H. rhamnoides extracts against microorganisms causing serious food poisoning and infections, namely E. coli, Salmonella typhimurium, S. aureus, Candida albicans, and Pichia jadinii were investigated in other studies which demonstrated their antifungal activity to be comparable with antifungal drugs – ketoconazole and mycostatin [Jeong et al., 2010].
Radish seed decontamination
In the study, the disinfecting effect of sea buckthorn extract and calcium hypochlorite was determined on radish seeds for sprouts inoculated with bacteria at a level of 106–107 CFU/g. The seeds were treated for 15 min, and the decontamination efficacy was additionally determined after soaking the seeds for 3 h. A reduction in the number of bacteria was found after 15-min treatment with both sea buckthorn extract and active chlorine (from calcium hypochlorite) (Figure 2A), while treatment with water, as a control, resulted in an increase in the bacteria number by 0.1 log CFU/g (E. coli, S. aureus), 0.2 log CFU/g (S. enterica), and 1.0 log CFU/g (L. monocytogenes). Contrary to the results presented above from the disc diffusion method, on seeds, the extract exhibited significantly higher antibacterial activity against E. coli, S. enterica and S. aureus than calcium hypochlorite (Figure 2A). The disinfecting effect of the extract ranged from 72.1% for S. aureus to 92.0% for E. coli, while for active chlorine these values were 21.3% for S. aureus and 70.7% for E. coli. The number of L. monocytogenes was reduced the most, by 93% after the treatment with the sea buckthorn extract and by 97.5% in the presence of active chlorine.
Figure 2
Effectiveness of radish seed decontamination after 15-min treatment with calcium hypochlorite and sea buckthorn fruit extract (A), and 3-h soaking of seeds in water or sea buckthorn extract (B). Means within individual strains followed by different letters differ significantly (p<0.05).
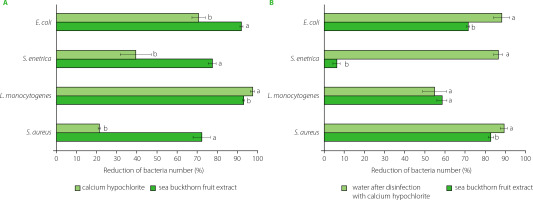
According to published data, the treatment of seeds with 20,000 mg/L calcium hypochlorite resulted in a variable reduction in bacterial counts of 1.0 to 6.5 log CFU/g [Beuchat et al., 2001; Montville & Schaffner, 2004]. Summarizing a broad review of the scientific literature, Montville & Schaffner [2004] demonstrated that 20,000 mg/L calcium hypochlorite treatment yielded a median reduction of 2.5 log CFU/g, although sometimes its efficacy was barely 0.5 log CFU/g or even less [Ding et al., 2013]. Putative factors contributing to such variability in results include different properties of various seed types. Barak et al. [2002] reported differences in the attachment and growth of S. enterica serovars and E. coli O157:H7 to alfalfa sprouts, which directly determine the disinfecting effect. As it has been shown, the adhesive properties of bacteria depend, among others, on roughness, wettability and porosity of seed surface [Fransisca et al., 2012; Zheng et al., 2021]. Furthermore, wrinkled seeds were not only characterized by much higher microbiological contamination but were more difficult to sanitize than the smooth ones [Charkowski et al., 2001].
As shown by Montville & Schaffner [2004], the efficacy of 20,000 mg/L calcium hypochlorite in reducing pathogen number depends on the duration of sanitization. Moreover, their analysis showed a linear correlation between inoculum size and microbial reduction. In the light of these findings, different sanitization efficacy can be expected depending on the type of seed, sanitization time and the level of seed contamination with bacteria, which also makes it difficult to compare and discuss published results. It should be noted that all the factors discussed above will also determine the activity of the sea buckthorn extract and the efficacy of disinfection conducted with its use.
In the study, the effect of decontamination of radish seeds varied significantly and differed after 3 h soaking process, with the seeds treated with calcium hypochlorite being soaked in water, and those treated with the sea buckthorn extract being further soaked in the same extract (Figure 2B). After soaking, the number of E. coli was reduced by 70.8% and 87.3% after the treatment with the extract and active chlorine, respectively. Interestingly, treatment and soaking in water resulted in a 0.7 log CFU/g reduction in E. coli counts, which may simply be due to the bacteria being washed off the seed surface.
In accordance with the recommendations of Codex Alimentarius Commission [2001] and European Sprouted Seed Association (ESSA) hygiene guideline for the production of sprouts and seeds for sprouting [ESSA, 2017], seeds should be thoroughly rinsed with water before the disinfection treatment to remove dirt and increase the efficiency of disinfection, and as appropriate, seeds should also be rinsed after the disinfection to eliminate its residues. The Commission also recommended a final water rinse of sprouts, which will remove the hulls, cool the product to slow down microbial growth and may reduce microbial contamination of the sprouts. Research carried out on germinated seeds washed in a stream of running water for 30 s showed that the number of microorganisms could be reduced in this way by a maximum of 1 log [Michalczyk & Kowalińska, 2009].
In the study, calcium hypochlorite was found to ensure a better disinfecting effect against E. coli, S. enterica and S. aureus after soaking the seeds than after a 15-min treatment, with a reduction of 87.3%, 85.5%, and 88.3%, respectively (Figure 2B). This may be due to the action of active chlorine, which remained on the seeds surface despite rinsing. Similar observations for hypochlorite used to disinfect rice seeds were reported by Miché & Balandreau [2001], who hypothesized that a chlorine coating was formed on the seeds, which could not be removed despite intensive rinsing. The possibility of removing bacteria from radish seeds during subsequent stages of rinsing, soaking and drying cannot also be excluded. However, in water as a control, the number of S. aureus increased by 0.5 log CFU/g and that of L. monocytogenes increased by 0.9 log CFU/g, while the number of S. enterica did not change after 3-h soaking in water.
In contrast to active chlorine, the seeds soaked in the sea buckthorn fruit extract resulted in lower decontamination efficiency than a 15-min treatment against E. coli, S. enterica and L. monocytogenes. A greater reduction after 3-h soaking in the extract was noted for S. aureus, and the number of bacteria was reduced by 81.9% (Figure 2B). After prolonged treatment, both substances also showed a much weaker sanitizing effect on the seeds contaminated with L. monocytogenes. The lower activity of the extract after 3-h soaking may result from bacterial cells being trapped in cracks or crevices of seed coat, which may additionally enlarge during the swelling and germination of seeds. According to Weissinger & Beuchat [2000], this phenomenon may limit direct exposure of pathogen cells to aqueous sanitizers and result in reduced efficacy of disinfectants.
Both L. monocytogenes and S. enterica are known to persist long-term in foods with low water activity, including seeds, in spite of the fact that their growth is not promoted in dehydrated products. However, during rehydration, bacteria can proliferate intensively, and their number may increase by 2.28 to 6.25 log CFU/g [Fay et al., 2023]. Also, during early stages of sprouting, the number of bacteria may increase by as much as 7–8 log CFU/g [Fransisca et al., 2012]. In this context, it seems that soaking seeds in sea buckthorn fruit extract may protect them against excessive and undesirable bacterial proliferation, even if its efficacy is lower than after 15-min seed treatment.
Influence of sea buckthorn extract and calcium hypochlorite on radish seed germination
For sprout producers, it is important not only to reduce the risk of development of pathogenic microorganisms, but also to identify the impact of the methods and substances used on seed germination ability and the related efficiency of the sprout breeding process. For this reason, the percentage of germinated radish seeds and the length of roots and hypocotyls of sprouts were also determined in the study. Compared to water treatment, the seeds treated with the sea buckthorn fruit extract showed significantly lower germination capacity after 24 and 48 h (Figure 3A). However, in the following days, the number of germinated seeds was comparable and amounted to 85.3% and 76.3% after 4 days, respectively. Whereas the number of sprouts obtained from the seeds treated with active chlorine was significantly lower than after the treatment with water or the extract. After 4 days, the number of germinated seeds was only 57.7%. A significant reduction in germination capacity after the treatment with calcium hypochlorite was also reported for alfalfa seeds [Beuchat et al., 2001; Kim et al., 2003].
Figure 3
Graphs of germination capacity of radish seeds (A) and the length of hypocotyls and roots of sprouts on the 4th day of cultivation (B), and photographic images of sprouts after treatment with water (C), calcium hypochlorite (D) and sea buckthorn fruit extract (E). Means followed by the different letters differ significantly (p<0.05).
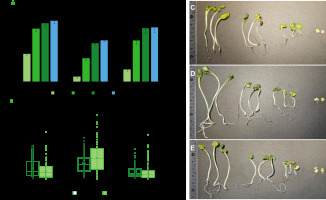
On the other hand, regardless of the seed treatment method, no significant differences were found in the length of roots and hypocotyls of sprouts (Figure 3B). This seems to be due to the large variation in the length of sprouts in population and the resulting large dispersion of the results obtained. However, the average values indicate similar parameters for sprouts from the seeds previously treated with water and extract, as well as longer hypocotyls and roots after seed treatment with active chlorine (Figure 3B-E). In the context of sprout breeding, however, uniform seed germination and sprout growth should be considered favorable.
Profile of phenolic compounds of sea buckthorn fruit extract
The water extract of sea buckthorn fruits contained 6 phenolic acids and 10 flavonoids from the flavonol group (Table 1). Among the phenolic acids, protocatechuic acid dominated, with its content in the extract reaching 5.43 mg/L. The second major acid was gallic acid (3.36 mg/L). The remaining acids were present at levels of approximately 1 mg/L or less. The dominant group of phenolic compounds of the extract were flavonoids including mono- and diglycosides of flavonols. Depending on their content, these compounds occurred in the sea buckthorn fruit extract in the following order: isorhamnetin 3-rhamnosylglucoside > isorhamnetin 3-rutinoside > isorhamnetin 3-glucoside > isorhamnetin 3-sophoroside-7-rhamnoside > isorhamnetin 3,7-diglucoside. The contents of the first three compounds mentioned were in the range of 12.99 to 3.41 mg/L. Other compounds whose contents in the extract were significant (above 1 mg/L) were quercetin 3-glucoside-7-rhamnoside and quercetin 3-sophoroside-7-rhamnoside.
Table 1
Content of phenolic compounds in the sea buckthorn fruit extract (mg/L).
Research on the phytochemical profile and the antioxidant and antiproliferative activities of sea buckthorn berries conducted by Guo et al. [2017], like our study, protocatechuic and gallic acids to be the major phenolic acids in terms of content. Taking into account flavonols, their contents were among the highest in Hippophae rhamnoides fruits [Guo et al., 2017; Wang et al., 2022]. According to Yang et al. [2009], sea buckthorn berries subsp. mongolica contained several times higher amounts of flavonol glycosides than in the case of chokeberry, cranberry, blackcurrant or bilberry. The most numerous flavonols identified in the sea buckthorn fruit extracts were isorhamnetin derivatives with one, two or three glycoside residues in the structure [Teleszko et al., 2015].
Previous investigations have indicated a discernible correlation between the antimicrobial activity of phenolic compounds and their structural attributes, notably pertaining to the configuration, quantity, and positioning of hydroxyl or methoxyl functional groups within the molecular framework [Gulcin, 2012; Wu et al., 2013]. Various studies have found that phenolic compounds can interact with bacterial cell walls [Bhattacharya et al., 2018; Tang et al., 2016; Yi et al., 2010; Zhang et al., 2015]. Phenolic compounds exhibit an affinity for bacterial cell walls, leading to perturbations in membrane integrity and subsequent efflux of intracellular constituents. Notably, Gram-negative bacterial species manifest a heightened resilience to phenolic compounds compared to Gram-positive bacteria. This phenomenon is intricately linked to the structural composition of their outer membrane, characterized by an abundance of phospholipids, imparting a formidable barrier against macromolecular ingress. The augmented resistance of Gram-negative bacteria to phenolic compounds may stem from the activity of enzymes localized within the periplasmic space. These enzymes are conjectured to catalyze the degradation of exogenously introduced molecules, thereby attenuating their cytotoxic effects [Efenberger-Szmechtyk et al., 2020]. However, there are also studies which suggest that phenolic compounds can cause the breakdown of the outer membrane of Gram-negative bacteria, leading to an increase in its permeability [Yi et al., 2010].
Research by Bhattacharya et al. [2018] showed that the polyphenol fraction of kombucha containing mainly catechin and isorhamnetin, as well as catechin and isorhamnetin themselves, have the ability to cause the permeability of the internal membrane of Vibrio cholerae. According to other researchers [Tang et al., 2016; Zhang et al., 2015], the damage to cell walls in S. aureus is probably caused by binding to the peptidoglycan layer, while in E. coli it is caused by oxidative stress. It is already known that phenolic compounds are able to induce endogenous oxidative stress in bacterial cells by inducing the formation of reactive oxygen species (ROS) [Tang et al., 2016; Zhang et al., 2015]. Damage to the cell wall may lead to the leakage of intracellular components. Plant extracts may cause an increase in intracellular protein leakage, depending on the concentration and incubation length [Tang et al., 2016; Zhang et al., 2015]. Furthermore, phenolic compounds have been empirically demonstrated to induce cytoplasmic leakage of various cellular constituents, encompassing nucleotides and ionic species, such as potassium and phosphate ions [Yi et al., 2010]. Furthermore, Dadi et al. [2009] showed that phe-nolic compounds (resveratrol, piceatannol, quercetin and its derivatives) were able to inhibit the activity of ATPase and ATP synthase in E. coli.
CONCLUSIONS
Sea buckthorn fruit extract showed varying antibacterial activity, and was more effective than the recommended 20,000 mg/L calcium hypochlorite in decontaminating radish seeds inoculated with E. coli, S. aureus and S. enterica. Soaking seeds in the extract may protect them against excessive and undesirable bacterial multiplication, although its decontamination efficacy was lower than after 15-min seed treatment. Moreover, the extract did not affect the germination capacity of radish seeds or the length of roots and hypocotyls of sprouts.
Sea buckthorn fruit extract can be used to reduce bacterial contamination of radish seeds prior to sprouting, additionally constituting a valuable source of phenolic compounds. Phenolic compounds determined in the extract have well documented health-promoting properties. However, further research is needed to investigate the extract’s impact on human health. Moreover, the use of the extract in seed sanitization requires microbiological quality control of both seeds and sprouts before they reach the consumer.
The demonstrated antibacterial effect of the sea buckthorn fruit extract allows recommending it as a natural product to be used to reduce bacterial contamination of seeds for sprouts without compromising their viability. The use of plant extracts as an effective means to increase the microbiological safety of sprouts fosters great potential in the food industry and represents a viable alternative to chemical antibacterial agents. However, further study is necessary to investigate strategies to prevent pathogen development during germination and sprout growth.