INTRODUCTION
Obesity is a form of chronic disease that is experiencing a rise in incidence and is now being recognized on a worldwide scale as an epidemic [Hill et al., 2022]. Recent research suggests that differences in lifestyle and eating habits are the most significant contributors [Khorshidian et al., 2021], and carbohydrate consumption, especially from refined sources, might lead to the development of chronic diseases including obesity, insulin resistance, metabolic syndrome, and diabetes [Stanhope, 2016]. Research evaluating the negative impacts of various forms of carbohydrates, including glucose, fructose, and sucrose, has found that fructose in sweetened foods and beverages has the most pronounced effect [Zafar et al., 2021]. Due to the fact that insulin cannot regulate fructose metabolism, it develops abdominal fat deposition that is distinct from overall obesity [Akar et al., 2021]. It is also well-known that a diet high in fructose causes insulin resistance, metabolic syndrome, diabetes, and heart disease, as well as serious damage to the liver, kidneys, vascular smooth muscle, ovaries, and testicles [Sumlu et al., 2022; Yildirim et al., 2019]. However, its impact on cellular signaling pathways may vary depending on the tissue type. For instance, hepatic insulin receptor substrate-1 (IRS-1), endothelial nitric oxide synthase (eNOS), protein kinase B (Akt), and sirtuin-1 (SIRT-1) levels were recently reported to be reduced in rats fed a long-term diet of 10% fructose [Korkmaz et al., 2019; Yildirim et al., 2019], whereas they were elevated in adipose tissues [Pektas et al., 2016]. This abnormality is due to insulin’s anabolic nature, which enhances adipose tissue growth. Furthermore, adipose tissue regulates various physiological processes via the hormones it secretes, including leptin, adiponectin, resistin, and visfatin [Liu et al., 2021], which may amplify the consequences of feeding-related metabolic disorders.
Insulin and insulin-like growth factor (IGF) signaling have been found to be associated with cell growth and division by promoting cell-cycle activators and inhibiting apoptosis-related pathways, which suggests a relationship between metabolic diseases and cancer development [Harvey et al., 2011]. There have been several postulated links between cancer development and obesity. For instance, increased levels and bioavailability of insulin and insulin-like growth factors, altered adipocytokine levels such as leptin, adiponectin, and visfatin, and oxidative stress have been associated with cancer development in obese people [Berger, 2014]. Epidemiological, clinical, and experimental studies also revealed the effects of weight loss in preventing breast, endometrium, esophagus, adenocarcinomas, pancreatic, colorectal, colon, and kidney cancers [Irigaray et al., 2007]. Recent studies present data revealing the alteration of insulin signaling components and apoptosis-associated parameters in high-fructose-induced obesity animals. For instance, caspase-3 levels were elevated [Savran et al., 2019], phosphorylated insulin receptor substrate (p-IRS-1) and phosphorylated protein kinase B (p-Akt), levels were lowered [Prasatthong et al., 2021], and insulin receptor (IR), IRS-1, IRS-2, and Akt were suppressed in the bladder of rats administered a high-fructose diet [Lee et al., 2021]. Caspase-3, -8, and -9 levels were elevated in the pancreas [Nephan et al., 2018], while tumor necrosis factor α (TNFα), B-cell lymphoma 2 protein (Bcl-2), Bcl-2-associated X protein (Bax), and caspase-3 levels were elevated in the liver [Zaki et al., 2019], and p53 levels were decreased in the colon epithelium of rats fed with high-fructose diets [Narayanankutty et al., 2022]. Similarly, we have previously demonstrated that levels of IR, IRS-1, IRS-2, Akt, phosphoinositide 3-kinase (PI3K), eNOS, and SIRT-1 in adipose tissue were enhanced [Pektas et al., 2016]. Even though these studies attempted to establish the molecular action mechanisms of fructose in various tissues, the effects of fructose on adipose tissue remain understudied.
Probiotics are a mixture of beneficial bacteria and/or yeasts that occur naturally in the human body, and when combined with prebiotics, they have been shown to treat a variety of ailments such as allergies, gastrointestinal problems, and inflammation [Markowiak & Śliżewska, 2017]. Kefir, a fermented milk product classified as source of probiotics, comprises lactic and acetic acid bacteria in a polysaccharide matrix [Rosa et al., 2016] and has been found to have anti-viral [Hamida et al., 2021], anti-inflammatory [Akar et al., 2021; Pektaş et al., 2022], and anti-allergic properties [Barros et al., 2021]. It has also been demonstrated to prevent problems brought on by fructose or high-fructose corn syrup (HFCS) ingestion [Akar et al., 2021; Chen et al., 2016; Ekici et al., 2022a; Rosa et al., 2016]. Also, the major bacteria in kefir, Lactobacillus kefiri, reduced the size of adipose tissue and had anti-inflammatory effects in fructose-fed mice [Zubiría et al., 2017]. Similar results were also seen in the adipose tissue of obese mice, where it reduced lipid production and inflammatory cytokines [Choi et al., 2017]. We have previously shown [Ekici et al., 2022a] that kefir protects young rats against the HFCS-induced inflammation of the masseter muscle and gingival tissue, as well as from the altered metabolic parameters that accompany this inflammation. We also showed the preventive effects on HFCS-induced bone loss in the craniofacial region of rat models [Ekici et al., 2022b]. Herein, we aimed to investigate the importance of apoptosis on adipocytes and adipogenesis, and the effects of kefir consumption on high-fructose corn syrup-induced adipogenesis at the molecular level.
MATERIALS AND METHODS
Animals and protocols
Male Wistar rats aged four weeks and weighing around 100 g were fed a regular rodent chow diet. Before the experiment, the rats were housed on a 12-h night and day cycle in an environment of 24–26°C and 50–60% humidity. The work was authorized by Afyon Kocatepe University’s Ethical Animal Research Committee (AKUHADYEK 49533702-55). The rats were cared in accordance with the National Health and Medical Research Council’s Experimental Animal Principles and Guidelines [National Health and Medical Research Council, 2013] and the National Institute of Health’s Experimental Animal Care and Use Guidelines (NIH issue no. 85-23, 1985 amended) [National Research Council, 2011]. A total of 48 animals were given a week to adapt to the environment before any practical applications were made, after which they were divided into four groups: control, kefir, HFCS, and HFCS+kefir. Kefir (DanemKefir, Isparta, Turkey) was supplied ready-made commercially available. Kefir was given to the rats as 1 mL/100 g body weight daily via gastric gavage. HFCS F55 (56% of fructose, 37% glucose, 2% higher saccharides, Cargill, Wayzata, MN, USA) was applied in drinking water (20%, w/v) ad libitum throughout 8 weeks. The terminal weights of the animals were 291±5 g (control), 322±3 g (kefir), 365±9 g (HFCS), and 335±2 g (HFCS+kefir). Following the administration of HFCS and kefir, the rats were rendered unconscious with ketamine (100 mg/kg) and xylazine (10 mg/kg), and then their omental white adipose tissues were removed and examined. Before being frozen using liquid nitrogen and kept at –85°C, the samples were washed with physiological saline solution. Some tissues were also fixed in 10% neutral formalin for the immunohistochemical analysis.
Determination of NF-κB and TNFα levels in the adipose tissues
Adipose tissue samples were homogenized in 0.1 M phosphate buffer (1:10 (w/v), pH 7.4) with Ultra-Turrax homogenizer (Ika Works Inc., Wilmington, NC, USA) at 24,000 cycles/min. Then, homogenates were further ultrasonicated at 20,000 cycles/s for 1 min (Dr. Hielscher ultrasonic device, Teltow, Germany). Supernatants were collected after centrifugation at +4°C at 10,000×g for 15 min and stored at –85°C until further use. Nuclear factor κB (NF-κB) and TNFα levels were determined using SEB824Ra and SEA133Ra commercial enzyme-linked immunosorbent assay (ELISA) kits, respectively (Cloud-Clone Corp., Houston, TX, USA) and performing the procedures outlined by the kit’s manufacturer.
Determination of protein levels by Western blot
Adipose tissues were homogenized using a Tissue RuptorTM homogenizer (Qiagen, Venlo, Netherlands) in 2-fold volumes of a homogenization medium (50 mM Tris, 150 mM sodium chloride, 5 mM ethylenediaminetetraacetic acid, 1% (w/w) Triton X-100, 0.26% (w/v) sodium deoxycholate, 50 mM sodium fluoride). The homogenates were then centrifuged at 1,500×g for 10 min at +4°C. Supernatants whose protein concentrations were determined by the Lowry method [Lowry et al., 1951] were collected and processed for Western blot analysis.
One hundred mg of proteins were separated on a polyacrylamide gel electrophoresis (12% separating and 4% stacking gels) and then transferred onto polyvinylidene fluoride membranes using the Trans-Blot Turbo transfer system (Bio-Rad Laboratories, Hercules, CA, USA). After that, the membranes were blocked with 3% bovine serum albumin for 1 h, and primary antibodies were utilized for priming the respective Akt (anti-Akt rabbit IgG, Santa Cruz Biotechnology, Dallas, TX, USA; dilution 1:1,000) and p-Akt (anti-pAkt rabbit IgG, Santa Cruz Biotechnology; dilution 1:500) antibodies overnight at +4°C. As an internal control, anti-GAPDH rabbit IgG (Santa Cruz Biotechnology; dilution 1:4,000) was used to label glyceraldehyde 3-phosphate dehydrogenase (GAPDH) for data normalization. Subsequent to washing, the blots were incubated with secondary antibodies conjugated with horseradish peroxidase (HRP) for 1 h (goat anti-rabbit IgG-HRP conjugate, Santa Cruz Biotechnology; dilution 1:10,000) before being exposed to a ClarityTM Western ECL (Bio-Rad Laboratories) substrate solution for 5 min. A ChemiDocTM MP chemiluminescence detection device was used to capture the blot pictures (Bio-Rad Laboratories) and ImageLab 4.1 software (Bio-Rad Laboratories) was used to determine the protein’s relative level in relation to GAPDH.
Determination of the gene expressions with the real-time polymerase chain reaction
Relative expression levels of genes: Akt, Nfkb, Tnfa, p53, Casp8, and Bcl2, with respect to Gapdh were determined with a semi-quantitative real-time polymerase chain reaction (qRT-PCR). To begin, total RNAs were extracted from adipose tissues using a commercial kit (GeneJET RNA purification kit, Thermo Fisher Scientific, Waltham, MA, USA) and then analyzed by agarose gel electrophoresis and a spectrophotometery at 260/280 nm for quality control. RevertAidTM First Strand cDNA Synthesis Kit was used to reverse transcribe 1 μg of total RNA to cDNA (Thermo Fisher Scientific). Gene expressions were determined by qRT-PCR protocol as we described previously [Ekici et al., 2022a]. The used primer pairs are given in Table 1. The relative expressions of the genes were determined using LightCyclerTM 480 SW 1.5.1 software (Roche, Forrenstrasse, Switzerland), with Gapdh expression serving as the internal control.
Table 1
Primer sequences used in gene expression analysis with quantitative real-time polymerase chain reaction.
Immunohistochemical analysis
Tissue slices (5 μm) were microwaved in a citrate buffer (0.1 M, pH: 6.0) for an antigen retrieval process after being deparaffinized and rehydrated. Hydrogen peroxide at a concentration of 3% was used to block peroxidase activity. To identify the desired protein, primary antibodies of NF-κB (sc-8008, 1/100), perilipin 1 (PLIN-1; bs-3789R, 1/200), Bax (sc-526, 1/100), caspase-3 (sc-56053, 1/100), and TNFα (ab34674, 1/200) were utilized. A secondary antibody coupled with horseradish peroxidase (HRP) was used to probe primed samples (TP-125-HL, Thermo Fisher Scientific). 3-Amino-9-ethylcarbazole (LabVision AB, Värmdö, Sweden) was applied as a chromogen. All of the slides were counterstained with Mayers hematoxylin and mounted using a water-based mounting solution. Under a bright light microscope (Eclipse E600, Nikon, Tokyo, Japan), the immuno-positive adipocytes were counted in 5 randomly chosen areas for each section to analyze the immunoreactivity of the specimens. Image analysis was carried out using imaging software (NIS Elements) specifically designed for that purpose (Nikon).
Terminal deoxynucleotidyl transferase dUTP nick-end labeling (TUNEL) assay
For the detection of apoptotic cells in adipose tissue, the indirect TUNEL method was used according to the manufacturer’s protocol of ApopTag plus Peroxidase in Situ Apoptosis Detection Kit (S7101, Merck Millipore, Burlington, MA, USA). TUNEL-positive cells were counted for each section in five different areas. Dark brown stained cells were considered positive.
Histochemical staining
Adipose tissue samples were fixed in 10% neutral formalin and histologically examined. Five micrometer-thick slices of paraffin blocks were cut and stained with hematoxylin and eosin (H&E). Five randomly selected areas from each section were evaluated from tissues of at least six rats. The average was used to compute the number of cells per unit. Furthermore, the diameter of at least 100 cells in each segment was measured, and the mean cell diameter was determined.
Statistical analysis
Throughout the course of the study, all of the data is given as a mean ± standard error of the mean (SEM). The one-way analysis of variance (ANOVA) and the necessary post hoc tests (Tukey) were used to conduct the statistical comparisons. Comparisons with p<0.05 were considered statistically significant. The expressions of genes and levels of proteins were standardized relative to the mean of the control group. The data was also normalized with corresponding GAPDH and Gapdh within the same groups.
RESULTS AND DISCUSSION
The information on the body and omentum weights of rats, their intake of food, fluids, and calories, as well as various metabolic characteristics, was published in our previous research [Ekici et al., 2022a]. Briefly, HFCS treatment significantly increased the body and omental weights of rats and dietary supplementation with kefir reduced the omental weights. HFCS-feeding resulted in an increase in plasma glucose, fructose, insulin, triglycerides, very low-density lipoprotein (VLDL) and total cholesterol, urea and uric acid levels. In turn, kefir administration to rats significantly lowered values of all these parameters.
In the current study, the effects of HFCS and kefir consumption on the Akt, p-Akt NF-κB, and TNFα proteins of the adipose tissue of rats were analyzed first. The results are depicted in Figure 1. Accordingly, HFCS-feeding increased Akt and p-Akt levels in rat adipose tissue while decreasing NF-κB, and TNFα levels (p<0.05 compared to control). Kefir administration in rats did not substantially alter Akt phosphorylation or inflammatory marker levels, although it did lower overall Akt levels (p<0.05). Kefir, when given to HFCS-treated rats, normalized the induction of total and phosphorylated form of Akt while simultaneously elevating levels of NF-κB, and TNFα in rats’ adipose tissues.
Figure 1
Western blot images for protein kinase B, Akt (A) and phosphorylated Akt, p-Akt (B), relative levels of Akt (C) and p-Akt (D) quantified using densitometry and normalized with corresponding glyceraldehyde 3-phosphate dehydrogenase (GAPDH), and levels of nuclear factor κB, NF-κB (E) and tumor necrosis factor α, TNFα (F) measured by ELISA in adipose tissues of rats from the control, kefir, high-fructose corn syrup (HFCS), and HFCS+kefir groups. Each band presented in a row (A and B) derived from the same experiment and blots were processed in parallel experiments. Values (C–F) are expressed as mean ± standard error of the mean. *Significantly different compared to the control group (p<0.05). #Significantly different compared to the HFCS group (p<0.05).
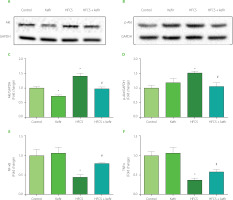
The levels of mRNA expression of Akt, Nfkb, Tnfa, p53, Casp8, and Bcl2 were determined using the qRT-PCR technique, and the findings are shown in Figure 2. The treatment with kefir had no significant influence on the levels of gene expression for apoptotic or inflammatory markers. In contrast, ingestion of HFCS led to an increase in the expression of the Akt and Bcl2 in the adipose tissues of rats, while at the same time leading to a decrease in the expression of the Nfkb, Tnfα, p53, and Casp8. In addition, the administration of kefir greatly returned all of the abnormalities that had been caused by HFCS.
Figure 2
Relative mRNA expression levels of Akt (A), Nfkb (B), Tnfa (C), p53 (D), Casp8 (E), and Bcl2 (F) of the adipose tissues of rats from the control, kefir, high-fructose corn syrup (HFCS), and HFCS+kefir groups. Data were normalized by Gapdh. Values are expressed as mean ± standard error of the mean (n=6–12). *Significantly different compared to the control group (p<0.05); #Significantly different compared to the HFCS group (p<0.05).
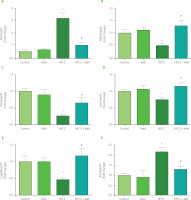
The images of adipose tissues of rats treated with HFCS and kefir after histochemical and immunohistochemical staining are given in Figure 3. The quantitative results of immunohistochemical analysis are summarized in Figure 4A–E. In comparison to the control group, the level of PLIN-1 in adipose tissues of rats consuming kefir was lower and the tissues of the group treated with HFCS had the highest PLIN-1 level (Figure 4A). It was also shown that the staining of PLIN-1 was less intense for the group that consumed HFCS+kefir compared to the group that consumed only HFCS. The NF-κB and TNFα levels, on the other hand, were higher in the adipocytes of kefir group (Figure 4B and 4C, respectively). When compared to the control group, NF-κB and TNFα levels were lower (p<0.05) for the HFCS group; however, the values for HFCS+kefir group were significantly (p<0.05) higher than for the HFCS group.
Figure 3
Images of histochemical and immunohistochemical staining of adipose tissues of rats from the control, kefir, high-fructose corn syrup (HFCS), and HFCS+kefir groups. H&E, hematoxylin and eosin staining.
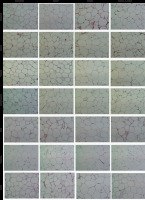
Figure 4
Relative intensity of immunohistochemical staining of PLIN-1 (A), NF-κB (B), TNFα (C), caspase-3 (D) and Bax (E) of adipocytes, number of TUNEL apoptotic cells (F) and number (G) and diameter (H) of hematoxylin and eosin (H&E) stained adipocytes of rats from the control, kefir, high-fructose corn syrup (HFCS), and HFCS+kefir groups. Values are expressed as mean ± standard error of the mean (n=6–12). *Significantly different compared to the control group (p<0.05); #Significantly different compared to the HFCS group (p<0.05).
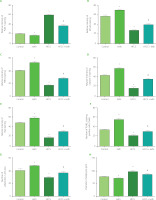
Figure 4F shows that the number of TUNEL-positive cells decreased significantly (p<0.05) in the HFCS group compared to the control group, and the number of apoptotic cells increased in the kefir group. Additionally, the number of apoptotic cells was higher in the HFCS+kefir group than in the HFCS group. On the other hand, the distribution of caspase-3 (Figure 4D) and Bax (Figure 4E) levels demonstrated a correlation with the number of TUNEL-positive cells. The levels of both markers were higher in the adipocytes of kefir group when compared to the control group, but they were shown to be lower in the HFCS group. In addition, an increase was found in the HFCS+kefir group in comparison to the HFCS group.
The changes in the number and diameter of adipocytes were evaluated with histological examinations under a light microscope. Results are shown in Figure 4G and H. It was found that the number of cells per unit area of tissues decreased while the cell diameter increased in the HFCS group as compared with control group. Besides, kefir administration to rats increased the average number of adipocyte but decreased the cell diameters. When administered to the HFCS-treated rats, kefir normalized the reduced cell number to the value of control group and decreased the cell diameters towards the value of the control group.
It is well known that consumption of processed foods that include fructose, glucose, sucrose, or any combination of these three in particular quantities can have negative consequences on human health [Stanhope, 2016]. Even though the effects of these dietary components on human health have been unequivocally demonstrated, the molecular mechanisms of their action continue to be the subject of debate. It has been demonstrated that an increase in dietary fructose intake lowers insulin sensitivity in liver tissue [Akar et al., 2021], but raises it in adipose tissue [Pektas et al., 2016]. Recent research indicates that feeding experimental animals with HFCS results in the development of omental obesity [DiNunzio et al., 2020; Hattori et al., 2021; Sadowska & Rygielska, 2019]. Despite this, there is still a great deal of confusion about the leading molecular mechanisms. As a result, the causes of possible adipogenesis due to the consumption of HFCS were investigated at the molecular level in this study. Additionally, the effects of milk kefir, which contains many bacterial species collectively referred to as beneficial bacteria, on possible changes were observed and analyzed in an effort to find a treatment.
What sets adipose tissue apart from other organs and tissues is its widespread distribution and its ability to enlarge in response to excess energy in the form of stored lipids. White adipose tissue and subcutaneous brown adipose tissue are the two primary forms of adipose tissue in animals, both of which surround the internal organs. Insulation and the storage of energy are the two primary physiological activities that are performed by adipose tissue, which are intimately related to the metabolic issues that are brought on by obesity, insulin resistance, and diabetes. In obese individuals, the expansion of adipose tissue may be mediated by hypertrophy, hyperplasia, or both processes. It is not yet known, however, how adipose tissue growth is regulated at the molecular level [Choe et al., 2016].
Recently, abdominal obesity due to long-term fructose consumption has been associated with increased insulin sensitivity due to enhanced level of Akt [Pektas et al., 2016], and decreased p-Akt levels in adipose tissues [Li et al., 2021]. However, augmented Akt and p-Akt levels in adipose tissues of mice fed a high-fat and high-fructose corn syrup diet for eight weeks were shown to be independent of plasma insulin levels [Zhang et al., 2020]. The proliferation of adipose tissue in fructose-fed rats was also associated with an increase in insulin sensitivity, in which Akt has an important role in the activation of the insulin signaling pathway [Maines et al., 2021]. Despite conflicting findings in the literature, this study provides evidence for Akt induction owing to HFCS intake at both gene and protein expression/phosphorylation levels. Besides, we also demonstrated the normalization of HFCS-induced Akt levels by kefir treatment. Furuno & Nakanishi [2012] demonstrated the suppression of antigen-induced mast cell activation with kefir through Akt inhibition, and Akt levels in rat kidney tissues were reduced with fructose and raised with the administration of Lactobacillus plantarum, which is the main beneficial bacteria in kefir [Korkmaz et al., 2019]. The usefulness of kefir ingestion in Akt regulation was obvious in HFCS-fed animals, even though study results vary depending on the tissue, duration, and dietary modeling.
Studies also indicated the function that TNFα played in the development of obesity-induced insulin resistance [Stephens & Pekala, 1992; Stephens et al., 1997]. Insulin resistance and the insulin signaling pathway have been found to be associated with TNFα and NF-κB. For example, changes in the level of TNFα in adipocytes can modulate the transcription of IRS-1 and Glucose transporter type-4 (Glut-4), both of which are involved in the transport of glucose [Barzilay & Freedland, 2003; Duncan et al., 2003]. A diet containing 10% fructose reduced TNFα and NF-κB protein level in adipose tissue while increasing their levels in the liver and kidney tissues [Qiao et al., 2018; Veličković et al., 2013] and modulated the tissue inflammatory states. Herein, our findings also demonstrated the suppression of TNFα and NF-κB levels by long-term HFCS-feeding while it also reduced the rate of apoptosis as evidenced by decreased apoptotic Casp8 and increased anti-apoptotic Bcl2 mRNA expressions in adipose tissues. Although similar studies in the literature have found that a high-fructose diet causes tissue inflammation [Akar et al., 2021; Korkmaz et al., 2019], the long-term use of HFCS appears to prevent apoptosis, leading to an increase in adipocyte survival.
Among the primary lipid droplet-binding proteins, PLIN-1 is abundantly expressed in adipocytes and its decreased expression leads to lower fat mass and insulin sensitivity. A recent study demonstrates that PLIN-1 deficiency promotes inflammatory responses and lipolysis in adipose tissue, resulting in insulin resistance [Sohn et al., 2018]. Increases in adipocyte diameters and sizes, as well as an increase in PLIN-1 level as measured by immunohistochemical technique in this study, are consistent with these investigations. However, results from TUNEL and H&E staining showed a reduction in both the number of viable adipocytes and the overall number of cells per unit area. The primary cause for this is the increase in the diameter of adipocytes that occurs in response to an increase in the number of cells that survive. In contrast to previous research [Pektas et al., 2016], the results of this study showed that omental obesity induced by fructose intake is caused by the suppression of apoptotic pathways in adipocytes. Considering the apoptotic potential of kefir and its constituent bacteria, a few studies have shown that kefir causes apoptosis in animal models of Ehrlich ascites carcinoma [Badr El-Din et al., 2020; Bozkurt et al., 2020; Esener et al., 2018] and enhances the apoptosis pathway [El Golli-Bennour et al., 2019]. Further, kefir was shown to have a modulatory role in initiating apoptosis and avoiding inflammation by lowering TNFα and NF-κB levels, as evidenced by the present study.
CONCLUSIONS
Our study results demonstrate that consumption of HFCS leads to adipogenesis via suppressing apoptosis and raising insulin sensitivity in adipocytes, in addition to the previously established link between the two. Although the clinical significance of these findings has yet to be confirmed, we showed that kefir might be a beneficial functional food against HFCS-induced abdominal obesity.