INTRODUCTION
The widespread acceptance of pasta is attributed to its low cost, ease of preparation, sensory attributes, and long shelf life [Kaur et al., 2013; Oliviero & Fogliano, 2016]. “Pasta” usually refers to various shapes and sizes of products made from a basic mixture of wheat (Triticum durum or Triticum aestivum) endosperm flour and water, cooked as fresh pasta or dried for consumption later on [Bresciani et al., 2022]. The ideal pasta characteristics include its smooth texture, translucence, hardness, brittleness, elasticity, as well as its rich amber color [Sicignano et al., 2015]. The nutrient composition of pasta is similar to that of the semolina used for its production, owing to which it has become recognized as a healthy food with low lipid content, no cholesterol, and a low glycemic index [Animashaun et al., 2017]. Moreover, the consumption of cereal products enriched with different plant materials that are rich in bioactive compounds has increased due to their positive influence on human health [Dziki, 2021]. As a result, the nutritional value of pasta has been increased by adding substances of an organic type or minerals [Wang et al., 2022].
Various ingredients such as tomatoes, spinach, basil, garlic, and flours or protein isolates from other grains and legumes have been incorporated into pasta formulations to enhance both its appeal and nutritional value [Kaur et al., 2013; Oliviero & Fogliano, 2016; Schmidt & de Oliveira, 2023]. Recently, the integration of plant-based products into pasta has been extensively tested, e.g., Padalino et al. [2014] examined the impact of adding up to 15% pea flour to spaghetti, finding a significant reduction in starch digestibility and optimal cooking time. Other studies have aimed to increase dietary fiber levels in pasta by adding high-fiber materials from sources other than durum wheat [Wang et al., 2022]. Pasta formulated with dietary fiber has shown a lower glycemic index than the non-enriched product and low responses in diabetics [Cui et al., 2024]. The addition of endoxylanases to pasta dough has been reported to reduce required extrusion pressure, decrease cooking time, lower dough hydration levels, and increase soluble fibers [Ingelbrecht et al., 2001]. The incorporation of smoked trout fillet powder enhanced the nutritional value of pasta, though it also increased cooking loss and decreased water absorption capacity and swelling index [Ozgoren & Yapar, 2019]. The combined use of propylene glycol alginate (0.1%), monoglycerides of fatty acids (0.5%), and gelatinization of mixed flour (buckwheat, corn, rice) has produced dried pasta with superior cooking characteristics and texture [De Arcangelis et al., 2020]. Bustos et al. [2019] investigated the effects of pasta enrichment with freeze-dried and air-dried blackberry, raspberry, and black and red currant powders on its antioxidant, sensory, and cooking quality. While the enriched pasta showed increased antioxidant activity, the enrichment negatively affected cooked pasta functionality, as evidenced by an increased swelling index. Enrichment with 50% of β-glucans and dietary fiber improved pasta cooking qualities, including stickiness, bulkiness, firmness, and the amount of total organic matter released in cooking water [Marconi et al., 2000]. Incorporation of barley β-glucans into pasta products resulted in a higher fiber content and lower glycemic responses [Yokoyama et al., 1997].
There is an increased demand for incorporating an inexpensive source of proteins that would justify the search for the potential utilization of protein-rich by-products as food [Schmidt & de Oliveira, 2023]. One such source may be sesame oil cake, a by-product of pressing oil from seeds, with a protein content of up to 40–50 g/100 g [Mostashari & Khaneghah, 2024]. Sesame protein provides essential amino acids necessary for human nutrition, supporting muscle growth, repair, and overall bodily functions. Moreover, sesame oil cake is abundant in dietary fiber, primarily cellulose and hemicellulose, which aid digestion and promote gastrointestinal health by regulating bowel movements and supporting beneficial gut bacteria [Wei et al., 2022]. Beyond proteins and fibers, sesame oil cake also contains notable levels of phenolic compounds, such as lignans [Mostashari & Khaneghah, 2024]. These compounds may help reduce oxidative stress, inflammation, and the risk of development of chronic diseases, such as cardiovascular ailments and certain cancers [Wei et al., 2022]. The presence of antioxidants, like tocopherols, further enhances the nutritional profile of sesame oil cake, contributing to its potential role in promoting overall health and well-being [Melo et al., 2021; Mostashari & Khaneghah, 2024]. Sesame oil cake further holds a notable economic value within the agricultural sector due to its dual role as a source of nutrients for livestock feed and as a potential ingredient in various industrial applications. These industrial applications not only contribute to the economic sustainability of sesame oil processing but also support environmental initiatives by promoting waste utilization. Therefore, the objective of this study was to evaluate the effects of using sesame oil cake as an additive ingredient on the pasta dough characteristics, processing ability, and stability of the end product.
MATERIALS AND METHODS
Materials
Semolina flour was obtained from a local mill (The Modern Flour Mills and Macaroni Factories Co., Amman, Jordan). Dried sesame (Sesamum indicum L.) oil cake, a by-product of pressing sesame oil, was purchased from Al-Nahda Pressing Mills Co., Amman, Jordan. Sesame oil cake was produced from the crop of 2022, a product of Sudan.
Flour blend preparation
Experimental flour blends were prepared by adding powdered sesame oil cake to durum wheat semolina at levels of 5, 7.5, 10, 12.5, and 15% (w/w) and mixing for 5 min using a medium-speed mixer (Pavan, Padova, Italy). The control flour was durum wheat semolina.
Proximate composition analysis
Moisture, ash, and protein contents of wheat flour, sesame oil cake and pasta products were determined using approved methods of the American Association of Cereal Chemists (AACC); methods no. 44-15.02, 08-01, and 46-13, respectively [AACC, 2000]. Crude lipid content was determined according to the AOAC International method 922.06 [AOAC, 2005], and crude fiber content was determined according to the International Organization for Standardization (ISO) standard method no. 5498.1981 [ISO, 1981], while nitrogen-free extract (NFE) was determined by difference. Analyses were carried out in duplicates.
Gluten content and gluten index determination
Gluten content and gluten index of flours were evaluated according to the AACC method 38-12.02 [AACC, 2000]. A 10.0±0.01 g sample was weighed and placed into the Glutomatic wash chamber (Glutomatic® 2200, Perten Instruments, Stockholm, Sweden) equipped with an 88-μm polyester sieve. Then, 4.8 mL of a 2% brine solution was added and mixed to form dough within 20 s. After the mixing period, the dough was washed for 5 min with water. To measure the gluten index, the wet gluten piece was centrifuged for 1 min at 2,415×g (centrifuge 2015, Perten Instruments). The fraction that passed through the sieve was scraped off and weighed, while the fraction remaining on the inside of the sieve was collected and added to the balance to obtain the total wet gluten content (g/100 g). For estimating dry gluten content (g/100 g), the total wet gluten piece was dried for 4 min at 150°C using a Glutork 2020 dryer (Perten Instruments). The gluten index (GI) was calculated using formula (1):
Results of five replicates were recorded for statistical analyses and means were calculated.
Falling number test
Flours were analyzed for falling numbers using the AACC method 56-81B [AACC, 2000]. A 7-g sample was combined with 25 mL of distilled water in a glass tube with a stirrer and shaken to form a slurry using Falling Number 1400 apparatus (Perten Instruments). The time (s) it took for the stirrer to fall through the paste was recorded as the falling number value. The measurement for each flour was performed in five repetitions.
Consistograph test
The consistograph test was conducted following the AACC method no. 54-50 [AACC, 2000]. The test was performed using the Chopin Consistograph (Tripette et Renaud, Asnières-sur-Seine, France) at both constant hydration (CH) and adapted hydration (AH) settings. The parameters determined were flour water absorption capacity (WA, %), hydration at 15% water (%), maximum pressure exerted by the dough against the mixer bowl lining (kPa), time needed to reach pressure maximum (s), tolerance time (s), and drop-in pressure at 250 s (D250, kPa) and 450 s (D450, kPa). The test was performed in five replicates.
Alveograph test
The alveograph test was conducted in accordance with the AACC-approved method no. 54-30A [AACC, 2000]. In brief, 250 g of wheat flour or flour blends were weighed, and then water (i.e., 60% of the flour weight) was added. After mixing the flour and water to form dough in a mechanical mixer for 8 min, the dough was allowed to rest for 20 min. After resting, the dough was divided into 150-g portions and was then shaped into smooth balls, and then flattened into a circular disc with a uniform thickness using a rolling pin. The shaped dough discs were then placed into a temperature-controlled chamber at 25°C, for an additional resting period of 30 min. After conditioning, the dough discs were placed onto the alveograph NG-97 (Tripette et Renaud) to run the test according to the manufacturer’s instructions. The alveograph test involved inflating the dough discs into bubbles, measuring and recording several parameters including dough strength (P; Pa), extensibility (L, mm), work (W, J), swelling index (G, the square root of the volume of air required to rupture the dough), configuration ratio (P/L), and elasticity index (Ie, %). The results of five tests were recorded for statistical analysis.
Pasta production
Pasta production was carried out in The Modern Flour Mills and Macaroni Factories Co., Amman, Jordan, using a pilot processing line for pasta (Pavan). Flours were mixed in the mixer of the pasta machine, and the water was added through an automatic sprayer to reach a final moisture content of 31±0.5 g/100 g. The mixing time varied from 10 to 12 min. The premix was then subjected to an extruder pressure of 11 MPa under a temperature of 45–50°C, and the dough sheet was formed with a moisture content of 29.5–30 g/100 g. Then it was passed through parallel sheeting rolls to obtain a stable thickness of 1.5 mm. The sheeted dough formed was cut by rotary cutting disks to reach a final length of 16 to 17 cm and a width of 6 to 7 cm. As a result, rectangular-shaped pasta dough was obtained.
The rectangular-shaped pasta dough was arranged on a mesh mounted on wooden frames, and the frame stacks were then pushed into a two-stage pre-drier. In the first stage of pre-drying, the air temperature at the entrance of the pre-drier was 50°C and increased gradually until it reached 56–59°C at the exit side. The relative humidity inside the pre-drier was in the range of 55–60%. The pasta’s moisture after the first stage was lowered from 29.5 to 22.0 g/100 g in 50 min drying time. During the second stage, the air temperature was increased to reach 70°C, while the relative humidity reached 75%. The moisture content of the pasta after the second stage of drying decreased from 22.0 to 18 g/100 g in 10 min time.
During the final drying stage, pasta sheets were exposed to three drying stages, which resulted in the production of pasta with a final moisture content below 12.5 g/100 g. After drying, the pasta product was cooled prior to the packaging process. The temperature of the cooling cabinet was around 30°C, the relative humidity was about 50%, and the total resting time was around 360 min. Identical pasta production protocol was replicated four times for each flour blend.
Pasta cooking gain, cooking loss, and cooking time evaluation
To evaluate cooking gain, 300 mL of distilled water were poured into and heated in a stainless-steel container covered with a cap circulated by cold water, and when the water started to boil, a 10-g sample of pasta was dropped in the boiling water to be cooked for different periods (10, 13 and 20 min). Cooking gain was evaluated by weighing pasta before and after cooking and expressed as an increase in cooked pasta weight (g/100 g) [Hummel, 1966].
To determine the amounts of solid substances lost in water after cooking (cooking loss), 30 mL of cooking water was placed in a small dry-weighed beaker and completely evaporated. After complete evaporation, the beaker was cooled in a desiccator and weighed to calculate the percentage of solid substances lost after cooking and expressed as g of matter loss per 100 g of dry pasta [Hummel, 1966].
Cooking time was measured by taking a small piece of pasta after being cooked in distilled water and crushed between two glass plates until the center white core could no longer be seen, and that indicated the optimum cooking time [Bergman et al., 1994]. Samples were taken after cooking for 10, 13, and 20 minutes and tested for their doneness states.
Pasta storage and acidity measurement
After processing, pasta samples (100 g) were packed in plastic bags, and stored inside an incubator (Memmert, Model No. INB, E212.0367, Rittersbacher, Schwabach, Germany) set at 25oC and 50% relative humidity. Samples were analyzed for acidity after zero, three, and six months. Acidity was measured according to the procedure described in Duszkiewicz-Reinhard et al. [1988] in which 10 g of ground pasta were weighed into a 250-mL beaker and mixed with 100 mL of 67% (v/v) aqueous ethanol. The extracts were titrated after filtration with 0.1 M NaOH, with phenolphthalein as an indicator.
Sensory evaluation
Five sensory experts of pasta quality from the Modern Flour Mills and Macaroni Factories Company in Jordan evaluated the quality of pasta products. Samples for sensory evaluation (pasta immediately after production and after 3 and 6 months of storage) were cooked to optimum cooking time in distilled water, drained, and served warm to sensory panelists. A score sheet was developed for judging the pasta samples according to their color, flavor, stickiness, and overall acceptability by using a hedonic scale test from 1 to 7, with 7 being liked very much and 1 being disliked very much [Duszkiewicz-Reinhard et al., 1988].
Statistical analysis
Data were analyzed using a SAS Institute [2003] software at the University of Jordan / Computer Center. Analysis of variance (ANOVA) of the different flours/doughs/pasta was performed using complete randomized design (CRD) using a t-test in which the rheological properties and chemical characteristics were used as a source of variation. The means of replicates from each variable were compared at a significance level of 5% using the least significant difference (LSD) test.
RESULTS AND DISCUSSION
Proximate composition of raw materials and end products
The proximate composition of semolina, sesame oil cake, and pasta produced from different formulations of these components is presented in Table 1. The ash content of semolina and sesame oil cake was 0.85 and 5.54 g/100 g, respectively. The pasta produced from flour blends containing different levels of sesame oil cake flour showed a significantly (p<0.05) higher ash content compared to the control pasta, with a linear increase as the rate of sesame oil cake addition increased. Pasta from the flour blends with 5, 7.5, and 10% (w/w) of sesame oil cake met the standard (no. 67) of the Jordanian Institute for Standards and Metrology [1996] for ash content in dry pasta (i.e., less than 1.20%), whereas those with 12.5% and 15% (w/w) of sesame oil cake addition had the ash content greater than 1.20%.
Table 1
Proximate composition of semolina wheat flour (S), sesame oil cake (SOC), and pasta from S (control) and blends of S with SOC.
Sesame oil cake addition to wheat flour resulted in an insignificant (p≥0.05) effect on pasta moisture content (Table 1). Moisture contents of semolina and sesame oil cake were 13.5 and 7.5 g/100 g, respectively. Table 1 also presents the protein content of durum wheat semolina, sesame oil cake, and pasta samples. Upon the addition of sesame oil cake flour to semolina, the protein content of the pasta products increased significantly (p<0.05) from 11.8 g/100 g for the control sample to 16.0 g/100 g for the pasta from the flour blend with 15% (w/w) of sesame oil cake, which corresponded to a 36% increase in protein content. A 17% increase in the protein content of pasta samples was accomplished with the addition of 10% (w/w) sesame oil cake flour. This was expected since sesame oil cake contained over 4.5 times more protein than semolina (54.2 and 11.9 g/100 g, respectively), which was reflected in the final product composition. A similar trend was obtained by Padalino et al. [2014], who reported that the fortification of wheat flour with pea flour considerably increased the protein, ash, and dietary fiber contents of the spaghetti samples when compared to the control sample.
In evaluating the nutritional benefits, one should consider the improvement in protein quantity as well as quality. Although it is no longer believed that plant proteins must be complemented at every meal, it is important to consume a wide variety of plant proteins to provide the body with an adequate supply of the limiting amino acids. Traditional protein combinations from various sources, such as sesame, soybeans, and wheat, can complement requirements for essential amino acids [Animashaun et al., 2017; El-Adawy, 1997].
The lipid contents of semolina and sesame oil cake were 1.19 and 18.31 g/100 g, respectively (Table 1). The addition of sesame oil cake to semolina showed a significant (p<0.05) increase in the lipid content of pasta samples from 1.13 g/100 g for the control to 3.21 g/100 g for the pasta produced from the flour blend with 15% (w/w) of sesame oil cake. This result was expected since the pasta has been enriched with lipids due to their high content in sesame oil cake, and this enrichment increased the lipid content as well as the nutritional value of the product.
The crude fiber content of semolina was 0.50 g/100 g and that of sesame oil cake was 2.00 g/100 g (Table 1). The increasing addition of sesame oil cake to semolina resulted in a gradual, significant increase (p<0.05) in the crude fiber content of the pasta samples, from 0.46 g/100 g for the control to 0.91 g/100 g for the pasta produced from the flour blend with 15% (w/w) of sesame oil cake. Yaseen et al. [2021] reported that the dietary fiber content of sesame seed is about four folds of its crude fiber. Therefore, the dietary fiber contents of the pasta from the flour blends with 10 and 15% (w/w) sesame oil cake are expected to be about 2.3 and 7 g/100 g, respectively, which represents an increase of 53% and 80% compared to that of the basic durum pasta products.
The nitrogen-free extract was 12.4 g/100 g for sesame oil cake and 72.0 g/100 g for durum wheat semolina (Table 1). As a result, adding sesame oil cake flour with its low nitrogen-free extract to semolina reduced the total nitrogen-free extract of the resulting pasta products from 76.9 g/100 g in the control to 69.6 g/100 g for the pasta samples from the blend with 15% (w/w) of sesame oil cake flour.
Quality parameters of wheat flour and flour blends
The wet gluten and dry gluten contents of flours used in pasta production are presented in Table 2. The increasing addition of sesame oil cake to semolina resulted in a significant decrease (p<0.05) in the wet gluten content of the blends that ranged from 28.8 g/100 g (semolina flour) to 22.7 g/100 g (flour with 15% (w/w) of sesame oil cake). These results were directly related to the dilution effect of gluten content due to the fact that sesame oil cake does not contain gluten. This observation agrees with that of El-Adawy [1997], who added different sesame products (i.e., sesame meal and sesame protein isolates) to the pan bread formulation. In fact, pasta made with a high legume flour content (>30%) is often characterized by poor cooking properties, such as high cooking loss, low swelling index, and cooking time, due to the dilution and weakening of the gluten matrix [Romano et al., 2021].
Table 2
Wet gluten and dry gluten contents, gluten index (GI), and falling number (FN) of semolina wheat flour (S) and its blends with sesame oil cake (SOC).
The dry gluten content of semolina was 9.5 g/100 g, and the significantly (p<0.05) lower values were found for the flour blends with an increasing proportion of sesame oil cake (Table 2). This reduction was parallel to the level of wet gluten content and indicates that the reduction of wet gluten is a true result of gluten drop rather than retardation of water absorption during gluten development.
Semolina had a gluten index of 89.9% (Table 2). The addition of 5% (w/w) of sesame oil cake to semolina significantly increased (p<0.05) the gluten index of the flour. However, this high value decreased significantly (p<0.05) for the flour blend containing 7.5% (w/w) of sesame oil cake and continued to decrease (p<0.05) for the flour with an increasing proportion of sesame oil cake. As a result, the gluten index of only the blend with 15% (w/w) of sesame oil cake was lower (p<0.05) than that of the control. This behavior may be due to a concentration-dependent interaction between sesame oil cake compounds and the gluten network. Gluten strength is widely recognized as an important factor for superior pasta cooking quality, though the relationship between gluten strength and pasta cooking quality remains complex [Matsuo, 1993]. Moayedi et al. [2021] found that the quantity of proteins, including gliadins, albumins, and globulins, as well as complex proteins, significantly influenced the variation in cooked firmness of fresh pasta.
The falling number values for semolina and semolina with 5, 7.5, 10, 12.5, and 15% (w/w) of sesame oil cake flour were above 400 s (Table 2), indicating that the addition of sesame oil cake to semolina samples at any level tested did not cause any significant difference (p≥0.05) in the falling number values. This result was expected since the sesame oil cake was processed from highly roasted sesame seeds in which all enzymes had been inactivated.
Rheological properties of pasta doughs
Consistograph test parameters
Data in Table 3 indicates that the maximum pressure decreased significantly (p<0.05) from 267.2 kPa for the control sample to 157.6 kPa for dough prepared from the flour blend with 15% (w/w) of sesame oil cake. No significant difference (p≥0.05) was observed only between dough from the flours containing 12.5% and 15% (w/w) of sesame oil cake. The maximum pressure decrease was attributed to the dilution effect of gluten through sesame oil cake, which causes weakening of the dough. Furthermore, it was expected that the increase in lipid content due to the addition of sesame oil cake may have caused dough softening through the lubrication and shortening action of sesame oil. Similar results were reported by El-Adawy [1997], who studied the farinograph characteristics of wheat flour bread supplemented with sesame proteins.
Table 3
Consistograph test characteristics of doughs made from semolina wheat flour (S) and its blends with sesame oil cake (SOC).
[i] All results are shown as mean ± standard deviation. Means in the same column with different letters are significantly different (p<0.05). PrMax, pressure maximum; WA, water absorption; HYDHA, hydration at 15% water; TPrMax, time needed to reach pressure maximum; Tol, tolerance time; D250 and D450, drop-in pressure at 250 and 450 s, respectively.
Generally, the addition of sesame oil cake flour resulted in a reduction in the water absorption values (Table 3). The water absorption decreased from 55.8% for the control dough to 50.9–52.7% for the samples from the flour blends. This reduction in water absorption was attributed to the high lipid content of the sesame oil cake flour. The higher lipid content made that less moisture was required than in semolina to achieve a similar dough consistency. Despite the different proportions of sesame oil cake in the flour blends the differences in water absorption between doughs were not significant (p≥0.05). This demonstrates that the addition of up to 5% (w/w) of sesame oil cake flour resulted in a weakening of the dough gluten matrix, whereas further additions were of minor effect. Padalino et al. [2014] concluded that the addition of pea flour considerably increased the cooking loss of spaghetti samples and caused a significant decrease in the swelling index and water absorption when compared to the control. On the other hand, Raungrusmee et al. [2022] reported that water absorption was considered an indicator of gluten network extension and gluten matrix maturation during dough formation.
The hydration at 15% water decreased from 53.3% for the control to 48.4% for the dough from the flour blend with 15% (w/w) of sesame oil cake, but no significant differences (p≥0.05) were detected between doughs with sesame oil cake flour content above 7.5% (w/w) (Table 3).
The time needed to reach maximal pressure ranged from 88.0 to 94.0 s and did not differ significantly (p≥0.05) among samples (Table 3).
Tolerance is the time during which the dough consistency stands within the pressure maximum and pressure maximum 20% range [Zheng et al., 2000]. Data in Table 3 indicates that the tolerance time value for the control dough was 122 s and that no significant differences (p>0.05) were found through the increasing addition of sesame oil cake except for the dough from the blend with 15% (w/w) of sesame oil case, in which a significant decrease (p<0.05) in the tolerance time was observed when compared to the control. Table 3 also presents the drop-in pressure from pressure maximum after both 250 and 450 s for the control sample of 78.7 and 87.5 kPa, respectively. A significant (p<0.05) decrease of drop-in pressure was presented with the addition of 5, 7.5, 10, 12.5, and 15% of sesame oil cake. The results agree with a study by El-Adawy [1997], who reported lower stability time with the addition of sesame products to wheat flour.
Sabanis et al. [2009] demonstrated that substituting wheat flour with gluten-free flours adversely affected the protein matrix during pasta processing, resulting in an unacceptable product quality. The texture of pasta made from wheat flours is directly related to the protein structure, particularly gluten proteins, due to their unique viscoelastic properties [Larrosa et al., 2016]. Additionally, the protein in sesame oil cake, which mainly consists of globulins [Kinsella & Mohite, 1985], is believed to weakly contribute to the texture of the wheat gluten network. The presence of fiber particles in sesame oil cake flour may also disrupt the protein-starch network stability, leading to lower firmness. Protein-starch interactions significantly impact the formation and strength of the protein gel matrix during dough network formation [Saleh, 2017]. Moreover, sesame seed proteins contain a high percentage of sulfhydryl groups that soften the dough structure [El-Adawy, 1997]. Taking the above into account, the results of the consistograph test in our study were attributed to the weakening effect resulting from higher levels of sesame oil cake addition. The results were in line with Filipović et al. [2017] who concluded that the incorporation of sesame in the flour formulation of pasta resulted in a reduction of the speed of starch gelation, which led to a reduction in starch network stability.
Alveograph test parameters
The elasticity values (P) determined for the doughs made from wheat flour and flour blends with sesame oil cake ranged from 392.3 to 500.1 Pa (Table 4). A significant (p<0.05) difference was only found between the control sample and dough from the blend with the highest proportion of sesame oil cake. This addition of sesame oil cake resulted in a decrease in the elasticity value. This may reflect an interaction process between the sesame components and the gluten network that also resulted in the dilution effect of gluten. In this regard, Barak et al. [2013] reported a positive correlation between P values obtained in the alveograph test and the glutenin content of the dough.
Table 4
Alveograph test characteristics of doughs made from semolina wheat flour (S) and its blends with sesame oil cake (SOC).
The extensibility (L) of all doughs containing sesame oil cake was significantly lower (p<0.05) when compared to the control dough (Table 4). The L values obtained after adding sesame oil cake flour up to 15% (w/w) were in the range of 53–64 mm, which is considered acceptable according to the CHOPIN protocol for durum wheat alveograph [Chopin Protocol, 2000].
The work (W) values, which represent the force necessary for the deformation of the dough, showed that the strength of the dough decreased significantly (p<0.05) with the increasing addition of sesame oil cake to the blend (Table 4). These results confirm the weakening effect due to the addition of sesame oil cake flour to durum semolina, and agree with a study by El-Adawy [1997], who reported that the addition of sesame cake weakened the bread formulation.
The configuration ratio represents the ratio of elasticity (P) of the dough to its extensibility (L). It was 0.63 for the control dough and increased significantly (p<0.05) in doughs with sesame oil cake (Table 4). The configuration ratio summarizes the viscoelastic behavior of the dough, and the results also confirm the weakening effect due to sesame oil cake addition to wheat flour. A configuration ratio between 0.5 and 1.0 was reported to provide an acceptable balance of extensibility [Tribune, 1999].
The effect of sesame oil cake on pasta cooking quality
At the optimum cooking time (10 min), the control sample showed a cooking loss value of 4.5 g/100 g dry pasta, which was significantly (p<0.05) lower than that of pasta from the blend with 7.5% (w/w) of sesame oil cake, but not significantly (p≥0.05) different than those of the other samples containing sesame oil cake flour (Table 5). However, the highest cooking loss, recorded for the pasta made from 7.5% (w/w) sesame oil cake blend (5.40 g/100 g dry pasta), did not exceed 0.9% of the result obtained for the control sample, which indicates that the pasta samples were in their acceptable cooking loss quality range. A cooking loss of 12 g/100 g or less was considered acceptable and indicative of good quality pasta [Fu, 2008]. Almost similar cooking loss trend was obtained for the pasta samples cooked for 13 and 20 min (Table 5). Again, the highest cooking loss values were determined for the pasta samples made with 7.5% (w/w) of sesame oil cake. The increase in cooking loss values of spaghetti fortified with edible legumes compared to spaghetti made from 100% semolina has also been reported by Filipović et al. [2017].
Table 5
Cooking gain and cooking loss of pasta made from semolina wheat flour (control) and blends of semolina (S) with sesame oil cake (SOC) and cooked for different times.
[i] All results are shown as mean ± standard deviation. Means in the same column with different letters are significantly different (p<0.05). Means in the same row (separately for cooking gain and cooking loss) were significantly different (p<0.05), and respective letters indicating significant differences were not shown.
The pasta cooking gain data is presented in Table 5. The cooking gain of pasta samples with sesame oil cake levels of 5, 7.5, and 10% (w/w) was significantly higher (p<0.05) compared to that of the control. The pasta produced from the flour bend with 7.5% (w/w) of sesame oil cake showed the greatest cooking gain when cooked for 10, 13, and 20 min. The control sample had a cooking gain of 198.0 g/100 g while the pasta made from semolina and 15% (w/w) of sesame oil cake blends had a cooking gain of 200.0 g/100 g at optimal cooking time (10 min). However, increasing the cooking duration for 3 or 10 min over the optimum (13 or 20 min) resulted in significant increases in the cooking gain values (p<0.05) for all pasta samples, which was attributed to giving starch granules an extra time to absorb more water. Results in Table 5 also show a significant decrease (p<0.05) in the cooking gain when the sesame oil cake proportion in flour blend was increased from 7.5% to 10% (w/w), indicating the optimal level of sesame oil cake affects the starch swelling process and the cooking gain. A significant increase in pasta cooking gain was also reported by Bergman et al. [1994] when they supplemented pasta with cowpea meal. In turn, Garcia-Valle et al. [2021] reported that the water adsorption capacity was higher in chickpea flour pasta (100.54%) than in durum wheat (59.32%) due to the higher content of dietary fiber in chickpea flour (15.88 g/100 g) as compared to durum wheat semolina (15.88 g/100 g), which might be behind the increased water adsorption capacity of the chickpea flour pasta.
Acidity of pasta during storage
The acidity of pasta produced from wheat flour and fortified with sesame oil cake flour during storage for up to six months is shown in Table 6. The volume of 0.1 M NaOH used to neutralize the control pasta at zero time was 1.64 mL. The acidity of pasta made using flour blends with 7.5, 10, 12.5, and 15% (w/w) of sesame oil cake recorded a significant increase (p<0.05) compared to the control, while the use of the blends with 12.5 and 15% (w/w) of sesame oil cake resulted in the highest acidity value of pasta.
Table 6
Acidity (mL of 0.1 M NaOH) of pasta samples made from semolina wheat flour (control) and blends of semolina (S) with sesame oil cake (SOC) during storage.
During storage, the acidity of each pasta increased significantly (p<0.05) (Table 6). The significantly (p<0.05) higher acidity was also found for the pasta samples made from flours containing sesame oil cake and stored for 3 and 6 months compared to the control sample. This increase can be attributed to the low-molecular-weight volatile fatty acids (secondary products of lipid autoxidation), which are extractable by the ethanol solution. However, the compact dry structure of pasta and the natural antioxidants of sesame oil may suggest limited oxidative rancidity. The presence of endogenous antioxidants in sesame oil cake may play a role in the stability of oxidation and contribute to maintaining nutritional quality and extending the shelf life [Melo et al., 2021]. Sesame seed oil is used in food industries as it has a longer shelf life and tremendous antioxidant properties [Yaseen et al., 2021], and sesame oil cake still contains a proportion of oil (32%) with a fatty acid pattern characterized by the abundance of unsaturated fatty acids [Melo et al., 2021]. Kinsella & Mohite [1985] concluded that sesame oil had excellent stability due to the presence of natural antioxidants such as sesamolin, sesamin, and sesamol. Our results agreed with those reported by Duszkiewicz-Reinhard et al. [1988], who fortified spaghetti with edible legumes.
Pasta sensory acceptability
Overall, pasta produced from wheat flour with 5, 7.5, and 10% (w/w) of sesame oil cake showed higher acceptability to the panelists than the pasta samples made from blends fortified with the higher contents of sesame oil cake, while pasta made from pure semolina recorded the highest acceptability (Table 7). Additionally, storage did not affect the panelists’ acceptability of pasta samples containing sesame oil cake or those produced only from wheat flour. There was a significant decrease (p<0.05) in the color-liking scores of pasta samples produced from flour blends. As the level of sesame oil cake in blended flour increased, the color of the pasta turned darker (Figure 1) and became less acceptable to the panelists (Table 7). No significant differences (p≥0.05) in the color scores were recorded between all pasta samples at zero time when compared to those stored for three and six months, which indicates color stability throughout the storage period.
Figure 1
Appearance of dry pasta produced from semolina wheat flour (control) and its blends with 5, 7.5, 10, 12.5, and 15% (w/w) of sesame oil cake (SOC).
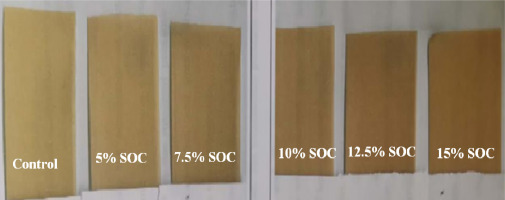
Table 7
Results of sensory evaluation using a seven-point hedonic scale of cooked pasta made from semolina wheat flour (control) and blends of semolina (S) with sesame oil cake (SOC).
The panelists showed a preference for the flavors of pasta produced from the flour blends containing 5, 7.5, and 10% (w/w) of sesame oil cake over pasta from the blends containing 12.5 and 15% (w/w) of sesame oil cake (Table 7). However, the control pasta received the highest flavor score. A similar observation was made by Bergman et al. [1994], who supplemented pasta with cowpea meal.
No significant difference (p≥0.05) was observed in the stickiness of cooked pasta from the flour blends containing 5, 7.5, and 10% (w/w) of sesame oil cake, while the pasta samples containing 12.5 and 15% (w/w) of sesame oil cake were significantly (p<0.05) stickier than the control sample (Table 7).
CONCLUSIONS
Results suggest the possible utilization of sesame oil cake in bakery products. The addition of sesame oil cake flour to semolina showed significant improvement in the pasta’s functional characteristics and nutritional value. The blend flours provided higher protein and lipid contents in the pasta; however, the sesame oil cake addition reduced the percentage of gluten content in the flour, thereby weakening the dough formulation and lowering water absorption capacity, which affected the final product’s quality characteristics. Moreover, the increase in cooking loss did not exceed 0.9% in any of the pasta samples containing sesame oil cake, which indicates that the pasta samples were in their acceptable cooking loss quality ranges. Sensorial evaluation of the flavor and stickiness of pasta produced from the flour blends containing up to 10% (w/w) of sesame oil cake was satisfactory and recommended to open the door for industrial utilization of such valuable and nutritious by-products. The storage period did not affect the panelists’ acceptability of pasta samples containing sesame oil cake.
Sesame compounds and gluten network interactions are believed to have a key role in modifying pasta’s functional characteristics. However, further investigation of sesame compounds and the gluten network interactions, including their effects on dough rheological characteristics and studying the protein quality of pasta fortified with sesame oil cake, is required to obtain higher quality pasta products.