INTRODUCTION
Sea fennel (Crithmum maritimum L., Apiaceae), also known as rock samphire or crest marine, is a halophytic species commonly found on cliffs and rocks, and somewhat less often on gravel or sandy soils along the Mediterranean, Black Sea, and European Atlantic coasts [Generalić Mekinić et al., 2016; Maleš et al., 2003; Meot-Duros & Magné, 2009]. The flowering period of the sea fennel usually extends from June to September and results in umbels with tiny yellow green flowers [Atia et al., 2011; Franke, 1982; Nartea et al., 2023]. It has been used for years as a source of vitamin C and in folk medicine as a diuretic, digestive and laxative [Franke, 1982; Renna, 2018]. Its essential oils (EOs) have the potential to be used in cosmetics, while the leaves are commonly used in cuisine either as a spice, brined or cooked [Radman et al., 2023; Renna et al., 2017; Renna & Gonnella, 2012]. The plant is also rich in carotenoids, minerals, organic acids, and phenolics with high biological activity [Generalić Mekinić et al., 2024; Maleš et al., 2003; Renna, 2018; Renna et al., 2017; Siracusa et al., 2011], especially substantial antioxidant and anti-inflammatory activity due to the high content of chlorogenic acid and its derivatives [Alemán et al., 2019; Generalić Mekinić et al., 2016, 2024; Meot-Duros & Magné, 2009; Nartea et al., 2023]. Due to its rich chemical composition and health-promoting effects, sea fennel has recently been recognised as a new “cash” crop for saline agriculture.
There are a large number of studies on the leaves [Generalić Mekinić et al., 2016, 2024; Houta et al., 2011; Kadoglidou et al., 2022; Martins-Noguerol et al., 2022; Nartea et al., 2023] and the aerial parts of the sea fennel [Alves-Silva et al., 2020; Nabet et al., 2017; Özcan et al., 2001, 2006], but only a few studies have investigated the flowers [Generalić Mekinić et al., 2016; Houta et al., 2011; Nartea et al., 2023; Politeo et al., 2023]. Moreover, these studies mainly focus on the EO profile and phenolic content, and contain little or no data on the other nutritionally and biologically important phytochemicals. Therefore, the main objective of this study was to investigate the chemical composition of sea fennel flowers from three different regions on the Croatian Adriatic coast. According to the results of previous reports, Croatian sea fennel could be considered as a specific chemotype; hence, this study aimed to gain an insight into the variability of chemical composition between populations from different geographical locations. The EOs as well as the FAs were analysed by gas chromatography-mass spectrometry (GC-MS), while the individual phenolic compounds and tocopherols were determined by high performance liquid chromatography (HPLC). In addition, the study included the determination of total phenolic content (TPC), total flavonoid content (TFC), and total tannin content (TTC) in the sea fennel flowers, and their respective antioxidant capacity. To the best of author’s knowledge, this is the first report on the presence and content of valuable antioxidant and nutritive phytochemicals in the flowers of the special chemotype of sea fennel from the Croatian coast.
MATERIALS AND METHODS
Chemicals, reagents and standards
All chemicals, reagents, and solvents used in this study were of analytical grade. HPLC grade acetonitrile and methanol were procured from Sigma–Aldrich GmbH (Steinheim, Germany), as were the phenolic HPLC standards, including chlorogenic acid (3-O-caffeoylquinic acid), cryptochlorogenic acid (4-O-caffeoylquinic acid), neochlorogenic acid (5-O-caffeoylquinic acid), caffeic acid, gallic acid, p-hydroxybenzoic acid, sinapic acid, ferulic acid, protocatechuic acid, and rutin. In addition, a series of n-hydrocarbons (C8–C40, Supelco), the standard set of fatty acid methyl esters (FAMEs) (Supelco 37 Component FAME Mix) and main reagents for spectrophotometric assays (Folin-Ciocalteu’s phenol reagent; vanillin; 2,4,6-tris(2-pyridyl)-s-triazine (TPTZ); 2,2-diphenyl-1-picryl hydrazyl (DPPH); fluorescein; 2,2’-azobis(2-methylpropionamidine) dihydrochloride (AAPH); and 6-hydroxy-2,5,7,8-tetramethylchroman-2-carboxylic acid (Trolox)) were also procured from Sigma-Aldrich. The tocopherols were purchased from LGC Standards Ltd. (Teddington, Middlesex, UK).
Plant material
The flowers of the sea fennel (C. maritimum L.) were used as plant material (Figure 1A). Sampling took place in early August 2022 along the Croatian Adriatic coast in the Dalmatia region. To cover the area of the Dalmatia region, samples were collected at three different locations: on the island of Pag (44°29’49.74’’ N, 14°54’58.19’’ E), the northernmost location, on the island of Korčula (42°57’7.80’’ N, 17°8’21.84’’ E) which was the most “pointiest” island in Central Dalmatia, and in the town of Cavtat (42°35’13.29’’ N, 18°12’41.90’’ E), the southernmost location (Figure 1B). About 500 g of fresh floral material was harvested for the study. The plant material for the analysis of all compounds, except for essential oil volatiles, was frozen and dried by lyophilisation using the FreeZone 2.5 system (Labconco, Kansas City, MO, USA), then homogenised in a hand mill and sieved through a 1 mm diameter screen. The obtained plant powder was used to extract the target compounds. For EO analysis, the plant material was air-dried at room temperature for 15 days and then used for oil extraction without any treatment.
Isolation and analysis of the essential oils
The hydrodistillation of sea fennel flowers was carried out for 3 h in a modified Clevenger apparatus containing a pentane and diethyl ether mixture (2:1, v/v) as an organic solvent trap. The detailed procedure was described by Bektasevic et al. [2023]. The isolated EOs were concentrated and stored at 4°C until analysis. The yield of extraction of EOs was calculated on a dry basis of the flowers (%, w/w).
The analysis of volatile organic compounds (VOCs) in the EOs was performed using a gas chromatograph (GC) equipped with an automated liquid injector and a tandem mass spectrometer (MS). Specifically, a model 8890 GC with a model 7693A liquid injector and a model 7000D GC/TQ mass spectrometer, all from Agilent Technologies Inc. (Santa Clara, CA, USA), was used for this purpose. VOC separation was performed on a non-polar HP-5ms column (5% phenylmethylpolysiloxane, dimensions: 30 m × 0.25 mm × 0.25 μm, Agilent Technologies Inc.) with helium as carrier gas at a flow rate of 1 mL/min. The injection volume was 1 μL at a split ratio of 1:50, and the inlet temperature was maintained at 250°C. The column temperature was programmed as follows: an initial isothermal phase at 60°C for 3 min, followed by a temperature gradient from 60°C to 246°C at a rate of 3°C/min, with a subsequent isothermal hold for 25 min. The MS detector operated at ionisation energy of 70 eV with an ion source temperature of 200°C. Ion scanning included a full scan mode ranging from 33 to 350 m/z.
Individual peaks were identified by comparing their retention indices (RIs) with those of a series of n-hydrocarbons (C8–C40) injected under identical conditions. In addition, software matching of mass spectra with commercial databases such as the Wiley 7 MS library (Wiley, NY, USA) and NIST02 (Gaithersburg, MD, USA), and comparison of mass spectra and RIs with literature data, aided in peak identification. The relative content of identified compounds was calculated as the percentage of the peak area. All analyses were conducted in triplicate, and results were expressed as mean ± standard deviation (SD).
Total phenolic, flavonoid, and tannin content determination
Extraction of the homogenised, freeze-dried sea fennel flower samples (0.5 g) was performed in a methanol and water mixture (80:20 v/v, 20 mL) by sonication at room temperature for 15 min followed by mixing in an orbital shaker for 3 h at room temperature. The samples were filtered (Chromafil™ Xtra PTFE syringe filter, 0.45 μm, Macherey-Nagel, Düren, Germany) after standing overnight in the dark at 4°C. The absorbance measurements in TPC, TFC, and TTC assays were conducted using a SPECORD 200 Plus, Edition 2010 (Analytik Jena AG, Jena, Germany).
The TPC of sea fennel flower extracts was determined using the Folin–Ciocalteu reagent [Singleton & Rossi, 1965] with results calculated based on a calibration curve for gallic acid and expressed as mg of gallic acid equivalents per g of dry plant matter (mg GAE/g d.p.m.).
TFC was determined by the colorimetric method using aluminium chloride [Yang et al., 2004], and the results were calculated based on a calibration curve for rutin, and expressed as mg of rutin equivalents per g of dry plant matter (mg RE/g d.p.m.).
TTC assessment was conducted using the vanillin-HCl method [Hagerman & Butler, 1994], with results calculated using a calibration curve for catechin and expressed as mg of catechin equivalents per g of dry plant matter (mg CE/g d.p.m.).
Each sample was measured in triplicate, and results were expressed as mean ± SD.
Individual phenolic compounds’ analysis
For the analysis of the individual phenolic compounds, the same extracts were used that had previously been prepared to determine the total content of phenolics, flavonoids and tannins. A Shimadzu Nexera HPLC system LC-40 (Shimadzu, Kyoto, Japan), equipped with a UV/VIS detector, was used according to the methodology described by Politeo et al. [2023]. Phenolic compounds were separated using a Phenomenex C18 reversedphase column (250 × 4.6 mm, 5 μm; Torrance, CA, USA). The column temperature (35°C) and flow rate (1.0 mL/min) were constant. The mobile phases consisted of an aqueous solvent (A) containing 0.2% o-phosphoric acid in water and an organic solvent (B) comprising methanol and acetonitrile in a 1:1 (v/v) ratio. The elution process started isocratically with 4% B for 5 min, followed by a gradient program: 5–16 min (linear gradient up to 15% B), 16–50 min (linear gradient up to 35% B), 50–62 min (linear gradient up to 4% B), and 62–65 min (4% B). Initial conditions were reinstated within 2 min and sustained for 10 min for column equilibration. Peaks were identified by comparing retention times with standards recorded under identical conditions, while quantification at 220 or 320 nm was conducted using calibration curves for external standards. Results were expressed as mg of phenolic compound detected per g of dry plant matter (mg/g d.p.m.). Two injections were performed for each sample, and results are expressed as mean ± SD.
Fatty acid analysis
To determine the composition of FAs in sea fennel flower samples, an analysis of FAMEs obtained by methylation was performed. Approximately 1 g of freeze-dried samples was mixed with 4 mL of 2-propanol and heated at 80°C for 15 min for extraction. Subsequently, 6 mL of n-hexane and 5 mL of a sodium sulphate solution (6.7%, w/v) were added, and the mixture was shaken vigorously. After centrifugation, the upper n-hexane layer containing the lipids was transferred to a new tube. The aqueous phase was extracted a second time with 7.5 mL of n-hexane and 2-propanol mixture (7:2, v/v), and the resulting upper n-hexane phase was combined with the previous extract. Subsequently, 3 mL of a methanol-toluene-sulphuric acid solution (88:10:2, v/v/v) was added to methylate the FA, and then the mixture was heated at 80°C for 1 h. The FAMEs were then extracted with n-heptane.
Analysis of the prepared FAMEs was carried out using a GC system (model 3900; Varian Inc., Lake Forest, CA, USA) equipped with a flame ionisation detector (FID), under the following conditions: a sample injection volume of 1 μL, an injector temperature of 250°C, and a split ratio of 1:50. Helium served as carrier gas with a flow rate of 2 mL/min. Separation was achieved using an RTX 2330 capillary column (30 m × 0.25 mm, 0.2 μm; Restek Corp., Bellefonte, PA, USA), with the detector temperature also set to 250°C. Samples were injected in duplicate, and a FAME standard set was injected under identical conditions to identify the compounds. The results of two injections (percentage of peak area) were expressed as mean ± SD.
Tocopherol determination
The tocopherols were extracted with n-hexane after saponification. First, 0.4 g of freeze-dried sea fennel flower powder was mixed with 1 g of ascorbic acid, 0.1 g of sodium sulphate, 20 mL of ethanol, and 4 mL of 60% potassium hydroxide solution. The mixture was heated in a water bath for 30 min at 85°C, shaking occasionally. After heating, the mixture was cooled, and 12 mL of water was added. A triple extraction was then performed by adding 10 mL of n-hexane. The resulting organic phases were combined, washed four times with 10 mL of water, dried at 35°C using a rotary evaporator, and finally dissolved in n-hexane [Knecht et al., 2015].
The analysis was performed using an HPLC system (Series 200, Perkin Elmer, Walthamn, MA, USA) equipped with a fluorescence detector (FLD). A volume of 20 μL was injected on an Ultra-silica column (250 × 4.6 mm, 5 μm, Restek Corp.). n-Hexane and 2-propanol mixture at a ratio of 96:4 (v/v) was used at a flow rate of 0.8 mL/min as the mobile phase to separate the compounds. The temperature of the column was maintained at 25°C. The excitation wavelength of 290 nm and emission wavelength of 330 nm were used for detection. The α-, β-, and γ-tocopherol standards were injected under the same conditions and their retention times were used to identify the tocopherols in the samples. The α-tocopherol calibration curve was used to quantify all tocopherols. The content of individual tocopherols in sea fennel flowers was expressed in mg per kg of dry plant matter (mg/kg d.p.m.). All analyses were performed in triplicate, and final results were expressed as mean ± SD.
Antioxidant capacity determination
The antioxidant capacity of sea fennel flowers was determined by three different methods: ferric-reducing antioxidant power (FRAP); 2,2-diphenyl-1-picrylhydrazyl (DPPH) assay; and oxygen radical absorbance capacity (ORAC). The extracts prepared as described in the subsection “Total phenolic, flavonoid, and tannin content determination” were used in assays.
In the FRAP method, the antioxidant activity involved the reduction of iron(III)-tripyridyltriazine (Fe(III)-TPTZ) complexes to iron(II) complexes. A volume of 10 μL of the extract was added to 300 μL of FRAP reagent prepared according to the procedure described by Benzie & Strain [1996]. The absorbance of the reaction mixtures was measured at 593 nm, and the FRAP results were expressed in μmol Trolox equivalents per g of dry plant matter of sea fennel flowers (μmol TE/g d.p.m.).
In the DPPH assay, a DPPH radical solution in ethanol was prepared to an initial absorbance of 1.2±0.02. Then, 10 μL of the extract was mixed with 290 μL of the DPPH radical solution, and the mixture was stirred. After a 60-min incubation period, the absorbance was measured at 517 nm [Čagalj et al., 2022; Skroza et al., 2015]. Results were expressed as μmol Trolox equivalents per g of dry plant matter of sea fennel flowers (μmol TE/g d.p.m.).
The ORAC assay, which evaluates the ability of antioxidants to inhibit oxidation induced by peroxyl radicals, was performed following a slightly adapted protocol by Prior et al. [2003] which was described in detail in our previous paper [Čagalj et al., 2022]. Results were expressed as μmol of Trolox equivalents per g of dry plant matter of sea fennel flowers (μmol TE/g d.p.m.).
All measurements were performed in triplicate in 96-well plates using a UV/Vis microplate reader (Synergy HTX Multi-Mode Reader, BioTek Instruments, Inc., Winooski, VT, USA).
Statistical analysis
The results of each determination were subjected to the analysis of variance (one-way ANOVA) with a Fisher’s least significant difference post-hoc test to show differences between sea fennel flowers from different locations. Differences were considered significant at p<0.05. Analysis was performed using Statgraphics Centurion-Ver.16.1.11 Software (StatPoint Technologies, Inc., Warrenton, VA, USA).
RESULTS AND DISCUSSION
Sea fennel flower essential oils’ composition
The EOs from dried sea fennel flowers from three locations (Pag, Korčula and Cavtat) were extracted by hydrodistillation and subjected to GC-MS analysis which resulted in 15 identified volatile organic compounds (VOCs) (Table 1). The EO extraction yield ranged from 0.19 to 1.87%, w/w (Table 1), and results achieved are in agreement with those of our previous study on EO extracted from sea fennel flowers, in which the yield was 1.35% [Politeo et al., 2023]. Other studies reported a high content of EO in sea fennel flowers compared to other parts of the plant (e.g., leaf or stems) [Generalić Mekinić et al., 2016; Pateira et al., 1999].
Table 1
Volatile organic compound (VOCs) composition of essential oils (EOs) of Croatian sea fennel flowers from three locations (Pag, Korčula and Cavtat), analysed by gas chromatography-mass spectrometry.
Compound | RI | RI (library) | Relative content (%) | ||
---|---|---|---|---|---|
Pag | Korčula | Cavtat | |||
α-Thujene | 931 | 930 | 0.12±0.01 | - | - |
α-Pinene | 938 | 939 | 3.17±0.93a | 1.90±0.33b | 1.80±0.72b |
Sabinene | 977 | 977 | 31.43±1.24a | 0.68±0.15b | 0.32±0.01c |
β-Pinene | 979 | 978 | 0.11±0.01 | - | - |
β-Myrcene | 993 | 994 | 0.15±0.01 | - | - |
α-Terpinene | 1,019 | 1,020 | 1.12±0.42 | - | - |
p-Cymene | 1,027 | 1,095 | 0.67±0.27b | 1.34±0.21a | - |
Limonene | 1,032 | 1,035 | 50.82±4.82c | 85.92±4.32b | 96.78±0.37a |
(Z)-β-Ocimene | 1,035 | 1,040 | - | 0.09±0.01 | - |
(E)-β-Ocimene | 1,042 | 1,051 | 0.17±0.01b | 1.12±0.57a | - |
γ-Terpinene | 1,062 | 1,064 | 7.08±1.43a | 8.93±3.14a | 0.51±0.02b |
(Z)-Sabinene hydrate* | 1,070 | 1,067 | 0.19±0.01 | - | - |
α-Terpinolene | 1,090 | 1,089 | 0.19±0.01 | - | - |
Linalool* | 1,098 | 1,104 | 0.16±0.01 | - | - |
Terpinen-4-ol* | 1,179 | 1,178 | 4.58±1.90 | - | - |
TOTAL | 99.96 | 99.98 | 99.41 | ||
EO yield (%, w/w) | 1.87 | 0.99 | 0.19 |
The detected compounds belong to monoterpenes and monoterpenoids. Limonene was the predominant compound in EOs from flowers from all locations and constituted from 50.82% (Pag) to 96.78% (Cavtat) of VOCs (Table 1). The lowest content of limonene was found in EOs from the sea fennel flowers collected in the northernmost location, while the highest content was found in EOs from the flowers collected in the southernmost location. The EOs from the flowers from the island of Pag, as the northernmost location, had the highest content of sabinene (31.43%), while its content in EOs of the flowers from the southern locations was quite low (0.68% in the Korčula sample and 0.32% in the Cavtat sample). Significant amounts of γ-terpinene were found in the EOs from the flowers from the island of Pag (7.08%) and the island of Korčula (8.93%), while it was detected in trace amounts (0.51%) in EOs of the flowers from Cavtat. The results of our previous studies on sea fennel leaves also showed variability in the EO profiles depending on the sites where the plants were collected from [Generalić Mekinić et al., 2024]. However, the major leaf EO compounds were also limonene (range from 24.3 to 93.2%), sabinene (range from 0.8 to 31.7%), terpinene-4-ol (range from 0.5 to 19.2%), and γ-terpinene (range from 0.5 to 8.7%) [Generalić Mekinić et al., 2024]. The Korčula sample had limonene as the major VOC, while the Pag sample had also high contents of sabinene and terpinene-4-ol, which is consistent with the findings reported for sea fennel flowers. Generalić Mekinić et al. [2016] analysed the composition of EO from dry sea fennel flowers collected in Split (Croatia) and demonstrated that limonene was the main compound (62.2%), followed by γ-terpinene (13.8%) and sabinene (12.0%). In the later study on Croatian sea fennel EOs from flowers, the contents of sabinene and limonene in samples from Central Dalmatia were almost equal (around 44%), while terpinen-4-ol (3.53%), γ-terpinene (2.79%) and α-pinene (1.76%) prevailed among the other compounds [Politeo et al., 2023]. Different results were reported for the EOs from dried flowers of sea fennel from Sicily, Italy [Pavela et al., 2017]. They contained 46.4% of γ-terpinene, 33.0% of thymol methyl ether, and 11.6% of p-cymene as main compounds. The other identified VOCs included α-pinene (accounting for 4.0% of VOCs), sabinene (1.0%), and carvacrol methyl ether (0.8%). γ-Terpinene (43.29%) was the predominant compound in EOs from fresh flowers of sea fennel grown in the garden of the Arid Land Institute (Medenine, southern Tunisia) [Houta et al., 2015]. Other important compounds were methyl thymyl ether (34.39%) and p-cymene (14.29%). Dillapiol was detected at 2.39%, while other compounds (α-thujene, α-pinene, sabinene, myrcene, phellandrene, terpinen-4-ol, thymol, α-zingiberene, and β-bisabolene) were present in amounts less than 1.0%.
Previous studies on the EOs of sea fennel confirm the presence of different plant chemotypes in the Mediterranean region due to the presence or absence of dillapiole in its EO [Pateira et al., 1999], and this study reconfirms that Croatian sea fennel does not contain it, so it can truly be considered a special chemotype.
Total phenolic, flavonoid, and tannin contents
The TPC, TFC, and TTC of sea fennel flowers from different locations are outlined in Table 2. The flowers from Cavtat, the southernmost location, had the highest TPC (26.21 mg GAE/g d.p.m.), while the lowest TPC was detected in the sample from the island of Pag (3.85 mg GAE/g d.p.m.). The TFC of the flowers collected on the island of Korčula was significantly (p<0.05) lower (0.51 mg RE/g d.p.m.) than that of the flowers from other locations (0.94–0.97 mg RE/g d.p.m.). The TTC of sea fennel flowers was in the range of 0.28–0.64 mg CE/g d.p.m. Our previous study [Politeo et al., 2023] reported higher content of all phenolic groups (total phenolics, flavonoids, tannins) in sea fennel flower extract than in the extracts of stems and leaves. However, Generalić Mekinić et al. [2016] analysed the TPC in the flowers, leaves, and stems of sea fennel, and found that the highest content of these compounds was found in the leaves (35.1 mg GAE/g), with slightly lower amounts in the flowers (32.6 mg GAE/g). Nartea et al. [2023] investigated the TPC of Italian sea fennel flowers and leaves, and the results ranged from 34 to 55 mg GAE/g depending on the harvest location. Interestingly, the highest TPC in their study was detected in the samples from southern Italy (Sicily and Apulia). A similar trend was also observed for TFC. Maleš et al. [2003] explored the phenolic content in the aerial parts of Croatian sea fennel at different flowering stages, and found that the samples before and at the beginning of flowering exhibited higher levels of total phenolics, flavonoids, and tannins than those at full flowering and fruiting stages. Houta et al. [2011] also compared the phenolic contents of different parts of the sea fennel. The sea fennel flowers were the plant parts with the lowest amounts of phenolics (TPC of 9.43 mg GAE/g; TFC of 3.71 mg CE/g; condensed tannin content of 0.43 mg CE/g).
Table 2
Total phenolic content (TPC), total flavonoid content (TFC), and total tannin content (TTC) in the Croatian sea fennel flowers from three locations (Pag, Korčula and Cavtat).
Location | TPC (mg GAE/g d.p.m.) | TFC (mg RE/g d.p.m.) | TTC (mg CE/g d.p.m.) |
---|---|---|---|
Pag | 3.85±0.08c | 0.94±0.14a | 0.28±0.09a |
Korčula | 20.43±0.06b | 0.51±0.05b | 0.40±0.05a |
Cavtat | 26.21±0.43a | 0.97±0.08a | 0.65±0.08a |
Phenolic compound profile
The HPLC analysis of the phenolics of sea fennel flowers from different locations resulted in the detection and quantification of ten compounds. Of these, nine were phenolic acids, and one was flavonoid (rutin) (Table 3). The contents of most individual phenolics were the highest in the flowers from Cavtat, which was consistent with the TPC (Table 2). Chlorogenic acid prevailed in all samples, and a significant (p<0.05) difference was found between the locations where the plant material was collected (Table 3). The content of this compound increased in the samples from north to south in a range from 0.30 (Pag) to 13.27 mg/g d.p.m. (Cavtat). Neochlorogenic acid, p-hydroxybenzoic acid, cryptochlorogenic acid and rutin were also found in considerable amounts in flowers from different locations. A somehow consistent pattern emerged, demonstrating the lowest content of individual phenolics in the sea fennel flowers collected at the northernmost site and the highest one in the sample from the southernmost location.
Table 3
Contents of phenolic compounds in Croatian sea fennel flowers from three locations (Pag, Korčula and Cavtat), analysed by high performance liquid chromatography.
Similarly to our research, Nartea et al. [2023] found that hydroxycinnamic acids were the main class of phenolics in Italian sea fennel with the highest content of chlorogenic acid, 34 to 53 mg/g, which are significantly higher than the results obtained in our study. Among the caffeoylquinic acids, the authors also identified neochlorogenic acid (1.5–2.3 mg/g), cryptochlorogenic acid (1.17–1.83 mg/g as) and 3,5-di-O-caffeoylquinic acid (41.0–57.7 mg/g). In our previous study, Generalić Mekinić et al. [2024] investigated the content of individual phenolics in sea fennel leaves from the same locations as in this study and reported the highest content of chlorogenic acid in the Korčula sample (248 mg/g d.p.m.), while the Pag sample had the lowest content of this phenolic compound (32 mg/g d.p.m.). A similar trend was also observed for neochlorogenic and cryptochlorogenic acids, with the highest contents of these phenolic acids found in the Korčula sample.
The content of chlorogenic acid in the Pag sample (Table 3) was consistent with the results of our previous study [Generalić Mekinić et al., 2016], in which it was determined at 7.7 mg/g in sea fennel flowers from Split, Croatia, which is geographically located south of the island of Pag and north of the island of Korčula. Meot-Duros & Magné [2009] analysed the phenolic compounds of sea fennel leaves collected from sand hills exposed to the sea spray and from the leaves below the cliffs, which were protected from the sea spray but also rich in organic matter. The phenolic compound content, especially chlorogenic acid, indicated that the plants located in a more stressful environment contained more phenolics. These observations can also be linked to the results of this study, considering the different climatic conditions of the Dalmatian north and the Pag site, which are characterised by notably lower annual temperatures and strong winds. These factors could indeed influence the synthesis and profile of phytochemicals in the plant.
Fatty acid profile
The FA profiles of Croatian sea fennel flowers are shown in Table 4. Of the 21 FAs identified, 11 were saturated fatty acids (SFAs), 2 were polyunsaturated fatty acids (PUFAs), namely linolenic acid (C18:3n3) and linoleic acid (C18:2n6), while 6 were monounsaturated fatty acids (MUFAs), namely pentadecenoic acid (C15:1n10), cis-7-hexadecenoic acid (C16:1n9), palmitoleic acid (C16:1n7), heptadecenoic acid (C17:1), oleic acid (C18:1), and eicosenoic acid (C20:1). To the authors’ best knowledge, this represents the first report of the FA profile in sea fennel flowers.
Table 4
Fatty acid profile of Croatian sea fennel flowers from three different locations along the Adriatic coast (Pag, Korčula and Cavtat).
Linoleic acid predominated (37.48%) in the Cavtat sample, while its content in the Korčula sample was 42.74%. In contrast, stearic acid (C18:0) predominated in the sample from the island of Pag with 31.29% (Table 4). Another four FAs (palmitic, oleic, linolenic and lignoceric acids) were detected in considerable amounts with relatively similar contents in the flowers from different locations. The differences in the dominance of the individual FA in sea fennel flowers collected at the different locations could be due to environmental factors. For example, it has been found that salinity increased the proportion of linoleic acid in sea fennel leaves [Ben Hamed et al., 2005].
The total content of SFAs in the samples ranged from 38.49% (Korčula) to 58.76% (Pag), that of MUFAs from 4.04% (Pag) to 6.34% (Korčula) and that of PUFAs from 37.23% (Pag) to 55.17% (Korčula) (Figure 2). SFAs predominated in the sample from the island of Pag, while PUFAs dominated in the samples from the island of Korčula and Cavtat. Although there are no studies on the FA profiles of sea fennel flowers, previous studies on leaves [Ben Hamed et al., 2005; Castillo et al., 2022; Labiad et al., 2021; Maoloni et al., 2021; Ventura et al., 2014] have shown that PUFAs were the major group of FAs, ranging from 33.8% to 86.0% of total FAs. MUFAs were the least represented group of FAs as in the samples of the current study. In turn, Generalić Mekinić et al. [2024] reported the highest content of palmitic acid in the Pag leaf sample (22.4%), which had also the highest content of SFAs (56.4%) and the lowest content of PUFAs (37.7%). The share of PUFAs in Korčula and Cavtat leaves was significantly higher, 44.2 and 46.4%, respectively, with the major acids being linoleic acid and linolenic acid.
Figure 2
Distribution of main groups of fatty acids of sea fennel flowers collected at different locations. SFAs, saturated fatty acids; MUFAs, monounsaturated fatty acids; PUFAs, polyunsaturated fatty acids.
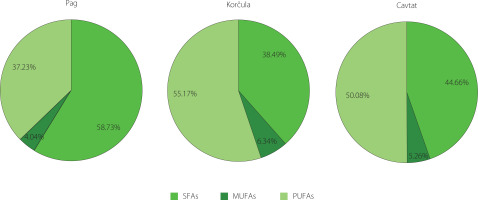
To sum up, our study results show the similarity of FA profiles between the samples from the southern regions as well as the specificity of the Pag sample.
Tocopherol profile
Tocopherols can occur in the form of four isomers: α-, β-, γ-, and δ-isomer. They play a protective role in plants [Havaux et al., 2005] but also contribute significantly to the prevention of certain chronic diseases and cancers [Huang et al., 2019; Xiong et al., 2023]. The analysis of sea fennel flowers collected from various locations along the Croatian Adriatic coast identified α-, β- and γ-tocopherol isomers, the profiles of which are shown in Figure 3. Furthermore, this is the first report on the tocopherols in the flowers of Croatian sea fennel, which – to the best of our knowledge – have not been investigated before.
Figure 3
Tocopherol (α-, β- and γ-) profiles of Croatian sea fennel flowers (mg/kg dry plant matter, d.p.m.) from three different locations along the Adriatic coast (Pag, Korčula, Cavtat). Different letters (a–c) above bars, separately for samples from each location, denote statistically significant differences (p<0.05).
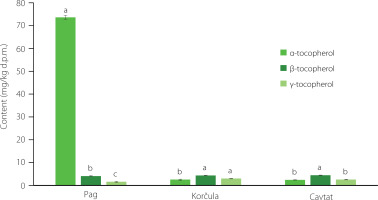
Similarly to the results described above (contents of EOs, phenolics, PUFAs), the flowers from the island of Pag were richer in tocopherols than the samples from the other locations (Figure 3). α-Tocopherol dominated (73.62 mg/kg d.p.m.) among tocopherol isomers in the Pag sample. In the other two samples, the content of α-tocopherol was more than 29 times lower, and β-tocopherol dominated, but with similar contents as in the flowers from the island of Pag. Our previous study on sea fennel leaves from the same locations and few others along the Adriatic coast showed the preponderance of α-tocopherol in the analysed samples (with its content ranging from 9.7 to 53.9 mg/g) [Generalić Mekinić et al., 2024]. This suggests not only the effect of the sampling sites on the profile of sea fennel tocopherols, but also the significant influence of the analysed morphological part of the plant. The content of tocopherols in wild and cultivated sea fennel from Italy was investigated by Nartea et al. [2023]. These authors also demonstrated the dominance of α-tocopherol (from 6.18 to 33.17 mg/kg d.p.m.), while reported the contents of β- and γ-tocopherols as the sum of these forms (from 0.93 to 3.33 mg/kg d.p.m.). When we compare these results with those of our study, it appears that the content of tocopherols in the Pag sample was more than 2-fold higher than in the richest Italian samples, while the results of the other two Croatian samples were lower than the value obtained for the Sicilian sample (the southernmost sampling point), which may also be related to the harvest geographical location [Nartea et al., 2023].
Antioxidant capacity
The effect of harvesting location on the antioxidant capacity of the sea fennel flowers was estimated by three antioxidant assays: FRAP, DPPH and ORAC (Figure 4). The FRAP was used to evaluate the flowers reducing potential, while the other two methods provided information on their radical scavenging capacity against synthetic DPPH radicals and peroxyl radicals (ORAC assay). The FRAP ranged from 7.37 μmol TE/g (Pag) to 99.74 μmol TE/g (Cavtat), and the ORAC ranged from 125.79 μmol TE/g (Pag) to 595.12 μmol TE/g (Cavtat). The highest ability to inhibit the DPPH radical was found for the sample from Cavtat but with similar result to the sample from the island of Korčula. The ability to scavenge the DPPH radical ranged from 0.63 μmol TE/g (Pag) to 2.14 μmol TE/g (Cavtat). The DPPH assay conducted for the extract of flowers collected in Split [Generalić Mekinić et al., 2016], which is geographically located between the islands of Pag and Korčula, showed that the ethanol extract of the flowers had a greater antioxidant potential than its EO. Pereira et al. [2017] investigated radical scavenging, ferric reducing and metalchelating activities of infusions and decoctions from stems, leaves and flowers of C. maritimum from Portugal, and reported the highest reducing activity obtained for the flower decoction and the activity against DPPH radicals for both flower samples (infusions and decoctions).
Figure 4
Antioxidant capacity of sea fennel flowers from different locations (Pag, Korčula, Cavtat) determined as (A) ferric reducing antioxidant power (FRAP), (B) 2,2-diphenyl-1-picrylhydrazyl (DPPH) radical scavenging activity, and (C) oxygen radical absorbance capacity (ORAC). Different letters (a–c) above bars denote statistically significant differences (p<0.05). TE, Trolox equivalent.
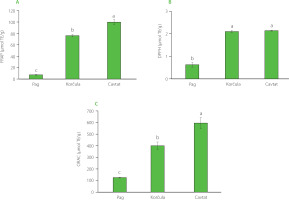
A comparison of the antioxidant potential (DPPH assay) of different parts of sea fennel was reported by Houta et al. [2011]. The authors found that the extracts from seeds and leaves had higher activity than the extracts from flowers and stems. On the other hand, Nartea et al. [2023] investigated the FRAP and free radical scavenging activity of sea fennel flowers and leaves from Italy, and reported the lowest antiradical activity of the flower extract from Sicily, but the highest reducing power of this sample. They also reported a promising antiradical activity of the samples characterized by a low TPC, but showing positive correlations with the content of chlorogenic acids. In addition, it should be taken into account that our previous study [Generalić Mekinić et al., 2018] on the antioxidant activity of sea fennel from different vegetation periods demonstrated variations in the activity of the extracts, and one of the weakest activities was detected for the August sample, which was also the harvest period in this study.
The chemical composition of sea fennel flowers, in this case the phenolic profile, was crucial for their antioxidant capacity. The flowers from Cavtat, which had the highest TPC and contents of chlorogenic, neochlorogenic and cryptochlorogenic acids, but also rutin, provided the highest antioxidant capacity, which could support the suggestion that these compounds were the main antioxidants in sea fennel flowers. As expected, the antioxidant capacity of the samples was correlated with the content of chlorogenic acids, which have been recognized as potent antioxidants [Miao & Xiang, 2020]. Chlorogenic acid is produced in plants during aerobic respiration from caffeic and quinic acid. It is widely distributed in plants, especially in green coffee beans, apples, aubergines, etc. [Wang et al., 2022].
CONCLUSIONS
In this study, the chemical composition and antioxidant capacity of the flowers of Croatian sea fennel were investigated. The results showed different chemical profiles depending on harvesting location. The northernmost sample from Pag had the lowest content of limonene and chlorogenic acids. The latter were mainly responsible for the high antioxidant capacity of the Korčula and Cavtat samples measured by DPPH, FRAP and ORAC assyas. However, the content of tocopherols, in particular of the α-isomer, was extremely high in the flowers from the island of Pag compared to the flowers from the other locations. It can be concluded that the samples from the northern, colder areas had a clearly different chemical profile and, accordingly, the antioxidant capacity, than the samples from the south. In general, however, despite these differences, sea fennel flowers can be considered a valuable source of various phytochemicals with great potential for further use in the food, pharmaceutical and cosmetics industries.